Your Guide to Triple Quadrupole ICP-MS Method Development
How To Guide
Published: June 20, 2024
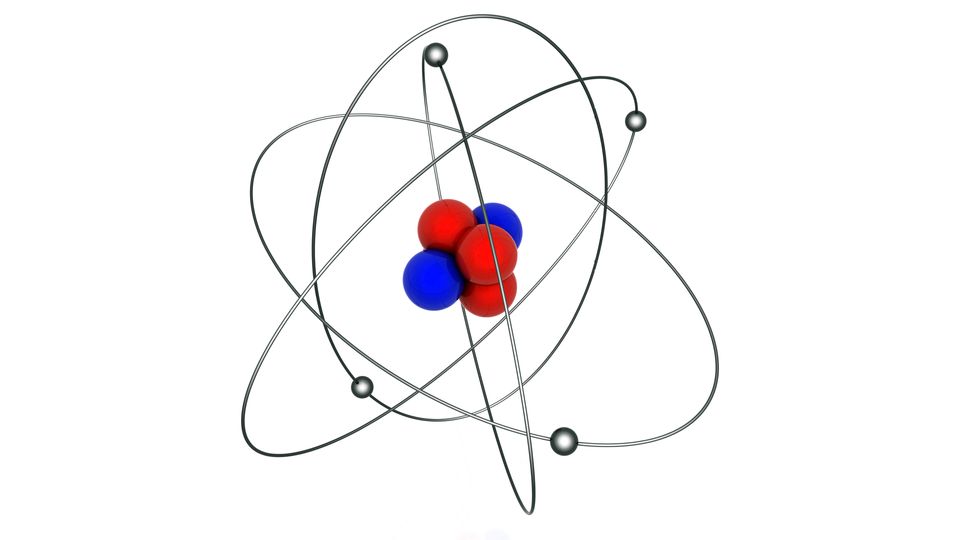
Credit: iStock
Inductively coupled plasma mass spectrometry (ICP-MS) is a key technique for the analysis of trace elements in a wide variety of sample types, owing to its outstanding speed and detection sensitivity.
By using the appropriate configuration for the sample introduction system, even challenging environmental samples can be analyzed. But biased or false positive results due to unresolved spectral interferences remain a cause of concern.
This guide explores the recent improvements in interference removal using triple quadrupole ICP-MS and the best solutions to analyze a full suite of elements in any sample matrix.
Download this guide to learn more about:
- How plasma conditions and sample matrix influence analysis
- Spectral interferences and how to overcome them
- Triple quadrupole ICP-MS method development for specific periodic elements
A comprehensive guide
to method development using
triple quadrupole ICP-MS
2
Introduction
Inductively Coupled Plasma Mass Spectrometry (ICP-MS) is recognized as a
key technique for the analysis of trace elements in a wide variety of sample
types. This is due to its outstanding speed, detection sensitivity and ability to
achieve detection limits generally in the low ng·L-1 concentration range for most
of the elements in the Periodic Table. For a number of elements, in particular
metals with low ionization potential and low natural backgrounds, detection
limits in the pg·L-1 range can be achieved, even under normal laboratory
conditions. Using a suitable configuration of the sample introduction system,
the introduction of even the most challenging sample types, such as soil digests
or undiluted sea water (total amount of dissolved solids approximately 3.5%),
is possible. ICP-MS is therefore considered the major technique for sensitive
and high throughput analysis. However, biased or false positive results are still
of concern for operators, lab managers and researchers, and in many cases,
the root cause is unresolved spectral interferences. This is especially true for
monoisotopic or quasi-monoisotopic elements, such as arsenic, which is a
key analyte in many regulated methods governing the safety of, for example,
drinking water, foodstuffs or pharmaceutical products.
After continuous improvement in interference removal through the use of
single quadrupole ICP-MS instrumentation equipped with a collision/reaction
cell (such as the Thermo Scientific™ iCAP™ RQ ICP-MS), recent years have
seen accelerated development of ICP-MS using triple quadrupole technology
(such as the Thermo Scientific™ iCAP™ TQ ICP-MS). These systems allow for
consistent interference removal regardless of the sample matrix. Developments
in hardware and software focusing on ease of use in routine trace elemental
determinations have allowed for triple quadrupole ICP-MS to be adopted by
increasing numbers of laboratories. Here, they are taking over analysis from
established techniques, such as Inductively Coupled Plasma Optical Emission
Spectroscopy (ICP-OES) and single quadrupole ICP-MS.
This compendium will show you the options and best solutions to analyze a full
suite of elements (non-naturally occurring elements excluded) in any sample
matrix, using triple quadrupole ICP-MS. For each element, a brief description
of the isotopes available for analysis as well as common and less common
interferences will be included. Finally, a table will highlight how each of the
elements can be successfully analyzed.
Example for the table format used throughout this document
A comprehensive guide to method development
using triple quadrupole ICP-MS
Element Barium
Available isotopes
130Ba (0.11%), 132Ba (0.10%), 134Ba (2.42%), 135Ba (6.59%), 136Ba (7.85%), 137Ba (11.23%), 138Ba (71.70%)
Ionization Potential 5.21 eV (10.00 eV)
Q1 resolution Intelligent Mass Selection (iMS)
Preferred analysis modes
Reaction Finder Default Alternatives
SQ-KED TQ-O2
(138Ba16 4 O)
2
1
The information shown in this compendium plus a wide variety of other,
additional possible reactions for all elements in the periodic table is stored in
the Reaction Finder method development assistant included in the Thermo
ScientitificTM QtegraTM Intelligent Scientific Data Solution Software and is
accessible anytime for consistent method development even for inexperienced
users. This includes optimized settings for all relevant parameters, such as
reactive gases, possible product ions (if any) and Q1 resolution.
1 All available isotopes with isocratic abundance are shown. The preferred
isotope is shown in bold.
2 The ionization potential indicates potential ion yield. Numbers in parentheses
indicate that double charged interferences can be formed by this element.
3 Resolution setting when triple quadrupole modes are used for analysis
4 Best and alternative choice for the analysis of a given element—potential
changes in product ions are mentioned in parentheses.
3
3
General conditions—how plasma conditions and sample matrix
influence the analysis
One of the reasons for the wide applicability of ICP-MS as a technique for
the analysis of trace elements is its high sensitivity combined with unique
robustness, enabling the analysis of a variety of sample matrices without (or at
least with reduced) matrix effects, in comparison to techniques such as organic
mass spectrometry. Matrix effects caused by a change of the sample matrix
still occur but can be reduced by choosing the right configuration of the sample
introduction system and compensated for by using suitable internal standards.
The matrix tolerance of ICP-MS is achieved as a result of the high plasma
temperature enabling full decomposition of the sample matrix to (mainly)
singly charged atomic ions, in the general form M+
. Every element with an
ionization potential lower than the first ionization potential of argon (15.76 eV)
should form ions in an argon generated plasma. However, the lower the
ionization potential of an element is, the higher is the degree of ionization, and
therefore the sensitivity. Some elements show 2nd ionization potentials well
below the ionization potential of argon, so that a significant formation of doubly
charged ions is observed, which in turn can lead to interferences on other
elements. Tuning the plasma to a high temperature allows rapid and effective
decomposition of the sample matrix leading to robust and reliable analysis of
complex sample matrices, such as food digests, drinking waters or soil digests.
The actual temperature of the plasma is impossible to measure, but generally
ranges between 6,000 to 10,000 K depending on the tuned conditions. For
the iCAP RQ ICP-MS and iCAP TQ ICP-MS systems, the typical plasma
temperature can be estimated to be between 7,500 and 8,000 K (using
the standard sample introduction system). Factors influencing the plasma
temperature include the RF power, the plasma gas flows, the size of the injector
tube etc. Also, the sample flow and the sample matrix play an important role, as
a too high sample flow or a complex matrix may consume a significant amount
of energy from the plasma and hence reduce the available energy for ionization
of critical elements, especially those with elevated ionization potential (≥ 8 eV).
The correlation between the degree of ionization and the ionization potential
for a given plasma temperature can be calculated using the so-called SahaLangmuir equation. Assuming a plasma temperature of 8,000 K, the following
ionization efficiencies are typically observed (Table 1).
The conditions of the plasma are often characterized by indicators such as
the formation rate of oxides (using for example the 140Ce16O+
/
140Ce+
ratio) and
doubly charged ions (using the M++/M+
ratio an element with a low 2nd ionization
potential, such as Ba or Ce). However, these two factors are closely dependent
on each other and it is not possible to tune an inductively coupled plasma to
reduce both of them at the same time. An increase in the plasma temperature
may help to reduce the amount of oxides formed, but it will increase the extent
of formation of doubly charged ions and vice versa. Although a higher plasma
temperature would allow for potentially higher sensitivity for elements with
elevated ionization potential, a higher formation of doubly charged ions, leading
to interferences, would be observed as well. Tuning (manual or automated) of
an inductively coupled plasma for mass spectrometry therefore takes both into
account. Generally, a well-tuned ICP-MS system achieves a formation of oxides
of typically less than 2% (1.5–2.0%), with doubly charged ions below 3.0% at
the same time (typically between 2.5 and 3.0%). As many elements form oxides
or doubly charged ions, a wide variety of interferences need to be overcome
because of their formation in the plasma.
Table 1: Dependency of the ionization efficiency from the ionization potential at a typical
plasma temperature of 8,000 K
Ionization potential Degree of ionization Examples
Below 6 eV 100% Alkaline and alkaline earth elements,
lower rare earth elements
Between 6–8 eV Close to 100% Transition metals, noble metals,
higher rare earth elements
Between 8–10 eV Decreasing to
about 50%
Some metals such as zinc, palladium,
cadmium, antimony, osmium, iridium,
platinum, gold.
Semi-metals and non-metals
(e.g., beryllium, boron, silicon,
arsenic, selenium, tellurium).
Higher than 10 eV Below 50 %
(10% @ 12 eV)
Mercury as the only metal. Non-metals
like carbon, nitrogen, oxygen, sulfur
and phosphorous, but also halogens
such as chlorine and bromine
4
Spectral interferences and how to overcome them
Spectral interferences commonly observed in ICP-MS include polyatomic ions,
doubly charged ions or isobaric interferences. In some cases, with very intense
signals observed on a mass adjacent to the analyte mass, an overlap of the
neighboring signal might be observed as well.
• Polyatomic interferences are formed through recombination of previously
ionized atoms as the ion beam traverses the interface region (e.g., between
sample and skimmer cone). Expansion into the vacuum (from atmospheric
pressure to about 2 mbar and subsequently to 10-7 mbar in the mass
analyzer) leads to cooling of the ion beam, and hence recombination
reactions can occur. Polyatomic interferences may be formed from all
elements present in the sample, but the most severe polyatomics are created
from argon ions in conjunction with O+
, and H+
derived from the aqueous
sample media, or if present, N+
, Cl+
, S+
derived from the acid matrix.
• Doubly charged interferences are formed through secondary ionization of
elements with particularly low ionization potential. As all mass spectrometers
detect ions on the basis of their mass to charge ratio (rather than mass alone),
they will impact analytes at half the mass of the interfering element. These
interferences are however easy to recognize in a mass spectrum.
Whereas polyatomic and doubly charged ions are formed in the plasma and
their signal level can, to some extent, be controlled by the plasma conditions,
isobaric interferences are caused by other elements present in the sample
sharing isotopes with identical mass number and cannot be influenced by
the instrument at all. However, in most cases they can be corrected for using
correction equations, provided that another isotope of the interfering element is
available and is itself free from interferences.
A common approach to interference removal is kinetic energy discrimination
(KED, Figure 1): Interference removal is achieved using helium in the collision
reaction cell. All ions traveling through the cell sustain collisions with helium,
although through its inert nature, no chemical reactions are induced. A positive
bias potential is applied between the CRC and the analyzing quadrupole,
so that only ions with sufficient kinetic energy remaining will exit the cell.
Polyatomic interferences will be efficiently suppressed as they have a higher
collisional cross section and lose more kinetic energy along the way. KED
is even more effective when used in combination with a low mass cut off to
eliminate ions of lower mass in the CRC, in order to reduce the formation of
additional interferences in the cell. KED is an effective tool for the removal
of polyatomic interferences, however, other types of interferences, such as
isobaric overlaps or doubly charged ions are not eliminated.
Triple quadrupole systems can also leverage KED in the same way as a single
quadrupole ICP-MS. In KED mode on a triple quadrupole system, the first mass
filtering quadrupole is operated as an ion guide only.
Figure 1: Interference Removal using Kinetic Energy Discrimination on the iCAP RQ ICP-MS.
He KED filters out
unwanted polyatomic
interferences, based on
difference in
cross-sectional size
of the analyte and
polyatomic
Low mass cut off
filters out precursor ions;
ions are then unable to
recombine later in the
QCell and backgrounds
are reduced further
40Ar+
, 40Ca+
, 35CI+
, 16O+ ArCI+
, Ca(OH)2H+
Quadrupole
isolates ions
required for
measurements
Comprehensive
interference
removal is
achieved
Target
Analyte
75As+
Complex
Sample
Matrix
5
Triple quadrupole ICP-MS systems such as the iCAP TQ ICP-MS achieve a new
level of data quality and confidence in the result, and hence allow a significant
improvement for key elements.
Interference removal based on triple quadrupole technology (also occasionally
referred to as MS/MS) includes a mass filtration step before the collision/
reaction cell, removing all ions of lower and higher mass than the analyte and
its interferences with identical mass to charge ratio. This initial mass filtration
allows reactive gases to be used for interference removal, which can induce
highly selective ion-molecule reactions between either the analyte or the
interfering ions with the gas. This will lead to a change of the ions observed,
and hence a second mass filtration step in the analyzing quadrupole will allow
the interference to be fully eliminated. The process of interference removal on
the iCAP TQ ICP-MS is illustrated in Figure 2:
The decision on whether a mass shift of the analyte (a so-called mass shift
reaction) occurs or whether the interfering ions will react (a so-called on mass
reaction) highly depends on the reactive gas used. Different gases exhibit
different reactivities to certain ionic species, in many cases forming simple
product ions, such as oxides (addition of an atom of oxygen), but also in other
cases, complex product ions. The latter is in particular observed with ammonia,
allowing very selective ion/molecule reactions with a variety of elements, but
also effectively reducing charged ionic species, such as some polyatomic
species. The following gases are typically used in conjunction with triple
quadrupole ICP-MS:
• Oxygen (O2): O2
induces a variety of effective chemical reactions with
different elements. For the most cases, an oxidation reaction will occur,
leading to a mass shift of the analyte (e.g., 75As will be converted into 75As16O).
This allows for effective suppression of polyatomic as well as doubly charged
ions. Differences in the reactivity towards oxygen can be used in order to
remove isobaric overlaps, such as the removal of 87Rb from 87Sr.
• Ammonia: Ammonia (NH3) can react in different ways. Whereas with some
elements, such as titanium, it forms a wide variety of reaction products (for
example [TiNH(NH3)x], it can also effectively eliminate polyatomic (especially
chlorine based) polyatomics. The removal of isobaric overlaps is as well
possible, for example, the elimination of 204Hg from 204Pb.
• Hydrogen: Although used for reduction of the 40Ar2
+
interference (affecting
the detection of 80Se), the use of hydrogen is not leading to a significant
performance improvement on triple quadrupole ICP-MS systems. Some
elements, such as chlorine or phosphorous, may react in a mass shift
reaction (to form ClH2
+
or PH2
/ PH4
+
), the yield is often low in comparison to a
mass shift reaction with oxygen. Nevertheless, for some applications, the use
of H2
can be beneficial.
In some cases, special analysis conditions can also help to improve the overall
performance of the ICP-MS analysis.
Figure 2: Possible reaction pathways in triple quadrupole ICP-MS.
Q3 set to product
ion mass (m/z 111)
Q3 set to product
ion mass (m/z 91)
Q1 set to analyte
mass (m/z 111)
Q1 set to analyte
mass (m/z 75)
Q2 filled with
reactive gas (O2)
Q2 filled with
reactive gas (O2)
MoO2
150 + Nd++, 150Sm++, 40Ar35Cl+
95Mo 150 + Nd+
, 150Sm+
, 91Zr+
95Mo16O+
forms
higher oxide
products such
as MoO2
75As+
is converted
into 75As16O+
111Cd 91 + [AsO]+
111Cd+
, 95Mo16O+
“On Mass”
75As+
, 150Nd++, 150Sm++, 40Ar35Cl+
“Mass Shift”
6
Out of the common toolbox for removing spectral interferences, there are a
number of techniques that can allow significant improvements, but may only be
applicable for a very limited set of sample matrices:
• Cold (or cool) plasma uses a reduction of the RF power from about 1500 W
to typically less than 700 W in order to reduce the plasma temperature and
hence the ionization of argon. Cold plasma conditions will typically favor the
ionization of elements with low ionization potential, especially alkaline and
alkaline earth elements such as Na, K, or Ca. In conjunction with the reduced
observation of argon based polyatomic species, a significant reduction
of backgrounds and detection limits is possible. However, cold plasma
conditions can only be applied for pure samples without any matrix load,
for example in the impurity screening of process chemicals in the
semiconductor industry.
• Adjustable quadrupole resolution settings can help to reduce the intense
signals obtained from the measurement of major components in a sample.
The resolution of the analyzing quadrupole can typically be reduced from
the standard setting of about 0.7 amu to about 0.3 amu almost instantly
during the analysis. In addition, an increase of the resolution setting allows
the negative effect of peak tailing of highly abundant signals adjacent to
an analyte, more commonly known as abundance sensitivity of the mass
spectrometric system, to be reduced.
Continue on to the next pages to learn more about how specific
elements can be analysed by ICP-MS
7
Periodic Table of elements
Click on any of the elements in red to jump to the specific
page to read more information on method development
using triple quadrupole ICP-MS.
8 Back to Periodic Table
Lithium, Beryllium, Boron
Elements with low mass rarely benefit from the use of a reactive gas, since
interferences are rare and interaction with heavier gas molecules (such as O2
or NH3) would lead to a significant reduction of sensitivity. Generally, these
elements can be analyzed using either standard (no cell gas mode) or KED
mode. Although there is a loss of sensitivity in KED mode for these low mass
ions, detection limits in the low ng·L-1 range are still achievable as backgrounds
are reduced as well. This enables a reduction of the overall run time for
multi-element analysis as there is no need to include an additional switch to
standard mode. Whereas beryllium is monoisotopic, both lithium and boron
have two isotopes, of which the heavier isotope in both cases has a significantly
higher abundance. The minor isotope of lithium is available as an enriched
stable isotope standard and is allowed for use as an internal standard for low
mass elements within a number of regulatory guidelines (such as EPA method
200.8). For boron, interferences can arise from the presence of organic solvents
(from peak tailing of the neighboring, very intense 12C signal) or in the presence
of high levels of Mg, (formation of 24Mg++ at mass 12 with tailing into 11B). The
use of a second mass filtration step using Q1 can improve the resolution of the
adjacent signal, even without the use of the CRC or any collision/reaction gas.
Element Lithium
Available isotopes 6
Li (7.50%), 7
Li (92.50%)
Ionization Potential 5.39 eV
Q1 resolution 1 amu
Preferred analysis modes
Reaction Finder Default Alternatives
SQ-KED SQ-N/A
Element Beryllium
Available isotopes 9
Be (100%)
Ionization Potential 9.32 eV
Q1 resolution 1 amu
Preferred analysis modes
Reaction Finder Default Alternatives
SQ-KED SQ-N/A
Element Boron
Available isotopes 10B (19.90%), 11B (80.10%)
Ionization Potential 8.30 eV
Q1 resolution 1 amu
Preferred analysis modes
Reaction Finder Default Alternatives
SQ-KED SQ-N/A, TQ-N/A
9 Back to Periodic Table
Sodium
Magnesium
Sodium is monoisotopic at m/z 23. Although no significant interferences are
expected, sodium is usually analyzed using KED mode (in e.g., environmental
or food samples) for attenuation of the high signals caused by its ubiquitous
nature. This can also be combined with increased resolution of the analyzing
quadrupole to further reduce the signal intensity and increase the dynamic
range even more. Where trace or ultra-trace analysis is required (for example
in the semiconductor industry) the use of cold plasma conditions can lead to a
significant improvement of detection sensitivity and limits of detection.
Magnesium has three isotopes, of which 24Mg is the default choice for analysis.
Although polyatomic interferences are unlikely to occur (with the exception of
12C2
+
formed when analyzing organic solvents), there may be a potential bias
caused by 48Ca++ in (e.g., environmental samples). Magnesium does show a
reduced reactivity to O2
compared to higher alkaline earth elements, but the
use of O2
followed by on mass analysis allows the aforementioned interferences
to be comprehensively removed.
Element Sodium
Available isotopes 23Na (100%)
Ionization Potential 5.14 eV
Q1 resolution Intelligent Mass Selection (iMS)
Preferred analysis modes
Reaction Finder Default Alternatives
SQ-KED SQ-N/A, TQ-O2
(23Na)
Element Magnesium
Available isotopes 24Mg (78.99%), 25Mg (10.00%), 26Mg (11.01%)
Ionization Potential 7.65 eV (15.03 eV)
Q1 resolution 1 amu
Preferred analysis modes
Reaction Finder Default Alternatives
SQ-KED TQ-O2
(24Mg)
10 Back to Periodic Table
Aluminum
Silicon
Aluminum is monoisotopic with its only isotope 27Al. This element may be
interfered in carbon containing solutions (arising from 12C14N1
H+
), but generally
it is not affected by significant overlaps. However, aluminum can show sample
matrix related errors, such as high signal fluctuation in the case of low sample
acidity, as a result of the formation of Al colloids under these conditions. Often
times, the addition of a small amount of acid can help to overcome this
potential issue.
Silicon is one of the most difficult elements for ICP-MS analysis, mainly because
of the ubiquitous nature of this element and the need to carefully control blank
levels. The major potential interferent is nitrogen from ambient air (forming an
14N2
+
interference on the major isotope of silicon, 28Si). Although other isotopes
of silicon are less interfered (29Si and 30Si), their low natural abundance limits
the attainable detection sensitivity. The choice of the most appropriate mode
for analysis strongly depends on the sample matrix. Whereas for aqueous
samples, the use of TQ-H2
(on mass reaction) allows to effectively suppress the 14N2
+
interferences, for organic solvents, the choice of TQ-O2
mode is superior.
Silicon can react with O2
to from 28Si16O, however, 12C16O as a potential interferent
would react likewise to form 12C16O2
with an equal m/z ratio. Therefore, detection
of 28Si16O2
+
is often providing superior results. Therefore, the best way for the
analysis of silicon is the use of H2
, leading to charge neutralization of the
polyatomic interferences, or, the use of the minor 29Si isotope in conjunction
with O2
.
Element Aluminum
Available isotopes 27Al (100%)
Ionization Potential 5.99 eV
Q1 resolution Intelligent Mass Selection (iMS)
Preferred analysis modes
Reaction Finder Default Alternatives
SQ-KED SQ-N/A, TQ-O2
(27Al)
Element Silicon
Available isotopes 28Si (92.23%), 29Si (4.67%), 30Si (3.10%)
Ionization Potential 8.15 eV
Q1 resolution 1 amu
Preferred analysis modes
Reaction Finder Default Alternatives
SQ-KED TQ-H2
(28Si),
TQ-O2
(28Si16O2
)
11 Back to Periodic Table
Phosphorous
Sulfur
Phosphorous is an important element due to its role in nutrition. It is also a
highly relevant element in life science applications, as it can act as a proxy for
DNA or DNA fragments, or be used for the detection and quantification of
post-translational modifications of proteins through phosphorylation. However,
due to its high ionization potential and significant polyatomic interferences
(principally 14N16O1
H+
), the analysis of phosphorous is often limited by reduced
detection sensitivity and elevated detection limits. Due to its monoisotopic
nature, there are no less interfered alternative isotopes available. However,
phosphorous reacts efficiently with O2
and hence the majority of polyatomic
interferences can be separated on a triple quadrupole ICP-MS system with
dramatically improved sensitivity.
Sulfur is one of the most difficult elements to be analyzed using ICP-MS.
Because of its high ionization potential, the ion yield is generally low, and
in aqueous media, there are highly abundant interferences, such as 16O2
+
.
Although the interferences on 34S are less pronounced (16O18O), the sensitivity
is also reduced considerably because of the lower abundance of the isotope.
The use of O2
and a mass shift reaction forming 32S16O can substantially improve
the detection limits (typically, sub ppb levels are achieved), but mass filtration is
essential to avoid interferences on the product ion mass through 48Ti or 48Ca.
Element Phosphorous
Available isotopes 31P (100%)
Ionization Potential 10.49 eV
Q1 resolution 1 amu
Preferred analysis modes
Reaction Finder Default Alternatives
TQ-O2
(31P16O) SQ-KED, TQ-H2
(31P1
H2
)
Element Sulfur
Available isotopes 32S (95.02%), 33S (0.75%), 34S (4.21%), 36S (0.02%)
Ionization Potential 10.36 eV
Q1 resolution 1 amu
Preferred analysis modes
Reaction Finder Default Alternatives
TQ-O2
(32S16O) SQ-KED (34S)
12 Back to Periodic Table
Chlorine
Potassium
Chlorine is an untypical element to be analyzed using ICP-MS. This is mainly
due to its high ionization potential leading to low ion yield from the plasma and
hence low sensitivity. In addition, hydrochloric acid is often an integral part of
sample preparation. Nevertheless, chlorine can be analyzed using ICP-MS,
for example to confirm its absence in process chemicals in the semiconductor
industry. The principal interferences on Cl are 17O2
1
H+
on 35Cl and 36Ar1
H+
on 37Cl,
with the latter interference being proportionately higher. The less interfered 35Cl
isotope is also the more abundant, so is preferred for analysis. The use of triple
quadrupole systems is beneficial for Cl analysis because of a positive effect
on sensitivity through ion focusing and acceleration in the CRC. Chlorine can
form product ions with both oxygen (e.g., 35Cl16O+
) and hydrogen (e.g., 35Cl1
H2
+
),
the latter being less effectively formed as two subsequent reaction steps are
required for its formation.
Potassium has its main isotope at m/z 39. Due to the adjacent and highly
intense signal caused by the main isotope of argon (40Ar), there may be a
contribution at low K levels from peak tailing. However, there is also direct, onmass contribution from 38Ar1
H+
. If the analysis of potassium at ultra-trace levels is
required, for example, in the semiconductor industry, cold plasma can be used
to reduce the formation of both 40Ar+
and 38Ar1
H+
ions and hence allow more
sensitive detection of K.
Element Chlorine
Available isotopes 35Cl (75.77%), 37Cl (24.23%)
Ionization Potential 12.97 eV
Q1 resolution Intelligent Mass Selection (iMS)
Preferred analysis modes
Reaction Finder Default Alternatives
TQ-O2
(35Cl16O) TQ-H2
(35Cl1
H2
)
Element Potassium
Available isotopes 39K (93.26%), 40K (0.01%), 41K (6.83%)
Ionization Potential 4.34 eV
Q1 resolution Intelligent Mass Selection (iMS)
Preferred analysis modes
Reaction Finder Default Alternatives
SQ-KED Cold Plasma
13 Back to Periodic Table
Calcium
Scandium
Calcium has several different stable isotopes with masses between 40 and
48. The most abundant isotope 40Ca is interfered through 40Ar and hence not
accessible when using hot plasma conditions. For typical applications, although
limited in abundance, the 44Ca isotope is used for analysis. The main polyatomic
interferences on this isotope are caused through 12C16O2
+
, 28Si16O+
. 27Al16O1
H+
or
32S12C+
, but there are also potential doubly charged overlaps with 88Sr++ or 89Y++.
As for potassium, cold plasma can be used to reduce the formation of 40Ar+
ions
and hence allow the analysis of the main isotope in cases where the sample
matrix concentration is low.
Scandium is very attractive as a potential internal standard for the transition
metals, as it is normally not present in most samples. However, its moderate
2nd ionization potential may lead to the formation of 45Sc++ ions, affecting the
detection of 23Na. Scandium can be interfered in the presence of phosphorous
(
31P14N+
) and silicon (29Si16O+
), which may lead to misinterpretation of system
stability when it is applied as an internal standard. Another potential influence
on scandium is zirconium, as its major isotope, 90Zr, may form doubly charged
interferences.
Element Calcium
Available isotopes
40Ca (96.94%), 42Ca (0.65%), 43Ca (0.14%), 44Ca (2.09%), 46Ca (0.004%), 48Ca (0.19%)
1st Ionization Potential 6.11 eV (11.87 eV)
Q1 resolution Intelligent Mass Selection (iMS)
Preferred analysis modes
Reaction Finder Default Alternatives
SQ-KED TQ-O2
(44Ca16O),
TQ-NH3
(40Ca)
Element Scandium
Available isotopes 45Sc (100%)
Ionization Potential 6.56 eV (12.8 eV)
Q1 resolution 1 amu
Preferred analysis modes
Reaction Finder Default Alternatives
TQ-O2
(45Sc16O) SQ-KED, TQ-NH3
(45Sc)
14 Back to Periodic Table
Titanium
Vanadium
Titanium has a remarkable isotope pattern with a total of five stable isotopes,
surrounding the most abundant isotope, 48Ti. Due to a potential isobaric
overlap with 48Ca, and polyatomic interferences caused by 31P16O1
H or 32S16O+
in
the presence of higher amounts of phosphorous and sulfur, respectively, the
preferred choice on a single quadrupole system is often 47Ti or 49Ti. However,
Ti reacts well with O2
and NH3. Whereas the use of oxygen alone would lead
to the formation of CaO+
, and hence would not remove the interference of Ca
(unless a combination of gases is used), titanium forms a variety of different
product ions with NH3, the most abundant of them typically being Ti[NH(NH3)
3]
+
and Ti[NH(NH3)
4]
+
, with mass to charge ratios of 114 and 131, respectively. If
calcium is present in the sample as well, the reaction with NH3
is therefore the
preferred choice as Ca reacts only slightly with NH3.
Vanadium has two stable isotopes, 50V and 51V, with 51V being significantly more
abundant. However, vanadium is strongly affected by interferences caused by
chlorine through the formation of 35Cl16O+
. This interference can be removed
using KED or the use of a reactive gas (O2
or NH3). Whereas O2
leads to a mass
shift (51V16O+
), the use of NH3
improves the removal of the ClO interference,
however, because V is not reactive towards NH3, it can be measured on mass
when this reaction gas is used. In presence of higher amounts of sulfur, the in
most samples unlikely formed 34S16O1
H polyatomic interference can become a
potential issue, fully removable through the use of triple quadrupole technology.
Element Titanium
Available isotopes
46Ti (8.00%), 47Ti (7.30%), 48Ti (73.80%), 49Ti (5.40%), 50Ti (5.50%)
Ionization Potential 6.83 eV (13.58 eV)
Q1 resolution Intelligent Mass Selection (iMS)
Preferred analysis modes
Reaction Finder Default Alternatives
SQ-KED TQ-O2
(48Ti16O),
TQ-NH3
(114[
48TiNH(NH3)
3])
Element Vanadium
Available isotopes 50V (0.25%), 51V (99.75%)
Ionization Potential 6.75 eV (14.66 eV)
Q1 resolution Intelligent Mass Selection (iMS)
Preferred analysis modes
Reaction Finder Default Alternatives
TQ-O2
(51V16O) SQ-KED, TQ-NH3
(51V)
15 Back to Periodic Table
Chromium
Manganese
Similar to vanadium, chromium has one major isotope (52Cr) and alternatives
with lower abundance. The main isotope is mostly interfered by 40Ar12,13C+
in
carbon rich sample matrices and, less heavily, by chlorine based interferences
(e.g., 35Cl16O1
H or 37Cl16O). Like vanadium, KED will efficiently remove the
polyatomic overlaps, whereas O2
and NH3
also allow the detection sensitivity
to be increased. When using O2
as a reactive gas, a mass shift reaction is
induced, whereas the element is measured on mass in conjunction with NH3.
Although monoisotopic manganese is not affected by a major argon derived
interference (40Ar14N1
H+
is relatively low and 40Ar15N⁺ is almost negligible due to
the low abundance of 15N), there are interferences that need to be considered
for certain sample types. In sample matrices containing higher amounts of
potassium, the detection of manganese may be affected by the formation of
39K16O+
. Samples containing elevated amounts of iron (such as whole blood) may
lead to peak tailing of the neighboring isotopes 54Fe and the more abundant
56Fe. Whereas the polyatomic interferences are easy to remove, the removal of
the peak tailing of iron will significantly benefit from the use of triple quadrupole
technology with either O2
or NH3
(as manganese is not reactive to either of the
gases), in combination with an increase of the resolution setting of the analyzing
quadrupole mass filter.
Element Chromium
Available isotopes 50Cr (4.35%), 52Cr (83.79%), 53Cr (9.50%), 54Cr (2.37%)
Ionization Potential 6.78 eV
Q1 resolution Intelligent Mass Selection (iMS)
Preferred analysis modes
Reaction Finder Default Alternatives
TQ-O2
(52Cr16O) SQ-KED, TQ-NH3
(52Cr)
Element Manganese
Available isotopes 55Mn (100%)
Ionization Potential 7.43 eV
Q1 resolution Intelligent Mass Selection (iMS)
Preferred analysis modes
Reaction Finder Default Alternatives
SQ-KED TQ-O2
, TQ-NH3
(55Mn)
16 Back to Periodic Table
Iron
Cobalt
Iron has four stable isotopes, of which 56Fe is the most abundant. However,
when analyzing iron on a single quadrupole ICP-MS, often the less abundant
isotope 57Fe is the preferred choice, since 56Fe is overlapped by an intense
polyatomic interference, 40Ar16O+
. This interference can be reduced using KED,
but signal to noise is often still favoring the alternative isotope. This overlap
however can be efficiently reduced when applying a reactive gas such as O2
or
NH3. In this case, the argon based polyatomic interference will be neutralized in
the cell, hence allowing the use of the most abundant isotope of iron.
Cobalt is monoisotopic at mass 59. Due to its low ionization potential and the
absence of any significant argon based polyatomic interferences, it can be
analyzed with high detection sensitivity using kinetic energy discrimination.
The presence of high amounts of nickel can lead to biased results through
peak tailing (58Ni and 60Ni surrounding 59Co) and 58Ni1
H+
formation, which can be
resolved through the use of a reactive gas or the use of high resolution settings.
Cobalt shows only a moderate reaction efficiency with oxygen, so an on mass
measurement is the preferred choice when selecting triple quadrupole based
modes.
Element Iron
Available isotopes
54Fe (5.80%), 56Fe (91.72%), 57Fe (2.20%), 58Fe (0.28%)
Ionization Potential 7.90 eV
Q1 resolution Intelligent Mass Selection (iMS)
Preferred analysis modes
Reaction Finder Default Alternatives
SQ-KED TQ-O2
, TQ-NH3
(56Fe)
Element Cobalt
Available isotopes 59Co (100%)
Ionization Potential 7.88 eV
Q1 resolution Intelligent Mass Selection (iMS)
Preferred analysis modes
Reaction Finder Default Alternatives
SQ-KED TQ-O2
(59Co),
TQ-NH3
(59Co)
17 Back to Periodic Table
Nickel
Copper
The main isotope of nickel is 58Ni, however, the preferred isotope is in many
cases 60Ni to avoid an isobaric overlap with 58Fe. Nickel is not seriously affected
through polyatomic interferences in the majority of typical sample matrices and
does not show extended reactivity towards the main reactive gases commonly
used in triple quadrupole systems. The main choice for analysis is therefore
the use of kinetic energy discrimination, but the use of ammonia may help to
improve detection sensitivity in an on mass measurement.
Copper has two isotopes, 63Cu and 65Cu. Whereas normally 63Cu is the default
choice, for the analysis of samples containing elevated amounts of sodium, the
selection of 65Cu may be beneficial, as 63Cu can be affected by the formation of
40Ar23Na+
. Whereas the reactivity towards O2
is limited, copper does form several
cluster ions with ammonia, however, on mass measurement is also possible.
Element Nickel
Available isotopes
58Ni (68.27%), 60Ni (26.10%), 61Ni (1.13%), 62Ni (3.59%), 64Ni (0.91%)
Ionization Potential 7.64 eV
Q1 resolution Intelligent Mass Selection (iMS)
Preferred analysis modes
Reaction Finder Default Alternatives
SQ-KED TQ-NH3
Element Copper
Available isotopes 63Cu (69.17%), 65Cu (30.83%)
Ionization Potential 7.73 eV
Q1 resolution Intelligent Mass Selection (iMS)
Preferred analysis modes
Reaction Finder Default Alternatives
SQ-KED TQ-NH3
(95[
63CuNH(NH3)])
18 Back to Periodic Table
Zinc
Gallium
Zinc has a total of five stable isotopes, with the default selection often being
66Zn due to the absence of isobaric overlaps. Zinc also has a slightly increased
ionization potential, so that ion yield is lower compared to other transition
metals (e.g., Co). Whereas the element is limited in its reactivity to O2
, a product
ion is formed with NH3
through the addition of one molecule of NH3
per atom
(e.g., 83[
66Zn(NH3)]). Higher amounts of sulfur present in a sample lead to the
formation of 32S2
+
and 32S34S+
, interfering on 64Zn and 66Zn, which may be an issue
in some environmental samples, such as waste waters or flow back solutions.
Gallium has two stable isotopes, 69Ga and 71Ga. The more abundant isotope can
be seriously biased if the sample contains barium (the main isotope of barium
is 138Ba and tends to form 138Ba++ easily). Polyatomic interferences are less
pronounced, although a significant overlap could be observed through 55Mn14N+
.
Gallium can be applied as an internal standard.
Element Zinc
Available isotopes
64Zn (48.60%), 66Zn (27.90%), 67Zn (4.10%), 68Zn (18.80%), 70Zn (0.60%)
Ionization Potential 9.39 eV
Q1 resolution Intelligent Mass Selection (iMS)
Preferred analysis modes
Reaction Finder Default Alternatives
SQ-KED TQ-NH3
83[
66Zn(NH3)]
Element Gallium
Available isotopes 69Ga (60.10%), 71Ga (30.90%)
Ionization Potential 6.00 eV
Q1 resolution Intelligent Mass Selection (iMS)
Preferred analysis modes
Reaction Finder Default Alternatives
SQ-KED TQ-NH3
19 Back to Periodic Table
Germanium
Germanium is often used as a potential internal standard in a variety of applied
applications, such as environmental analysis. Due to severe polyatomic and
isobaric interferences on the major isotopes of germanium (e.g., 40Ar32S+
on 72Ge,
74Se+
on 74Ge and Cl2
+
on both 72Ge and 74Ge), it is most commonly analyzed
using a minor isotope, 73Ge. If potential contributions from selenium can be
excluded or are acceptably corrected through mathematical correction, 74Ge
is an appealing alternative due to its significantly higher abundance. Although
the use of triple quadrupole technology would remove the isobaric interference
of selenium on 74Ge, the resulting sensitivity when using NH3
is often lower as
compared to KED. However, germanium is also an analyte of high interest in the
semiconductor industry and therefore needs to be analyzed at very low levels in
process chemicals, such as different acids or organic solvents. Often, the use
of triple quadrupole technology is superior over cold plasma as germanium has
a relatively high first ionization potential.
Element Germanium
Available isotopes
70Ge (20.50%), 72Ge (27.40%), 73Ge (7.80%), 7
74Ge (36.50%), 76Ge (7.80%)
Ionization Potential 7.90 eV (15.94 eV)
Q1 resolution Intelligent Mass Selection (iMS)
Preferred analysis modes
Reaction Finder Default Alternatives
SQ-KED TQ-NH3
(89[
73Ge(14N1
H2
)]),
TQ-O2
(73Ge)
20 Back to Periodic Table
Arsenic
Arsenic is monoisotopic at mass 75. Due to its toxicity, it is a mandatory
element in many regulated methods. The most common interferences on
arsenic are chlorine based, such as either 40Ar35Cl+
or 40Ca35Cl+
, which can be
efficiently reduced using KED. Additional interferences such as 150Nd++ or 150Sm++
can be observed when rare earth elements are present. This is often the case
for soil samples, but food samples are also likely to be affected. The optimal
way to resolve these interferences is the use of oxygen in the collision / reaction
cell (leading to the mass shift formation of 75As16O+
). Arsenic can also be present
in a variety of different chemical forms, or species, varying dramatically in
toxicity. The element is therefore a primary example for the need of speciation
analysis in order to obtain information on the exact species present in a sample
rather than the total concentration. Although potential interferences would
be separated as well in the chromatographic separation, the use of triple
quadrupole technolgy improves the overall sensitivity, enabling the detection of
especially low abundant (and eventually otherwise missed) species.
Element Arsenic
Available isotopes 75As (100%)
Ionization Potential 9.82 eV
Q1 resolution Intelligent Mass Selection (iMS)
Preferred analysis modes
Reaction Finder Default Alternatives
TQ-O2
(75As16O) SQ-KED
21 Back to Periodic Table
Selenium
Selenium has six stable isotopes with varying abundance. The most abundant
isotope, 80Se, is not the preferred choice due to the 40Ar2
+
interference derived
from the argon plasma. On single quadrupole instruments, the preferred choice
is therefore a less abundant, but less interfered isotope, such as 77Se, 78Se, or
82Se. In addition, bromine can interfere on 80Se and 82Se through the formation
of 79Br1
H+
and 81Br1
H+
. Like arsenic, doubly charged ions of certain rare earth
isotopes, such as 156, 160Gd, can cause doubly charged interferences on the main
Se isotopes. As with arsenic, the optimal way to resolve these interferences
is to use O2
in the cell to mass shift Se to SeO+
. This approach enables use of
the most abundant 80Se isotope, leading to drastically improved sensitivity and
lower detection limits when using a triple quadrupole based ICP-MS instrument.
Element Selenium
Available isotopes
74Se (0.86%), 76Se (9.23%), 77Se (7.60%), 78Se (23.69%), 80Se (49.80%), 82Se (8.82%)
Ionization Potential 9.75 eV
Q1 resolution Intelligent Mass Selection (iMS)
Preferred analysis modes
Reaction Finder Default Alternatives
TQ-O2
(80Se16O) SQ-KED (78Se),
SQ-H2
(80Se)
22 Back to Periodic Table
Bromine
Like all halogens, bromine has a high 1st ionization potential, leading to low
ion yield in an inductively coupled plasma. Nonetheless it can be analyzed
with good detection limits. Bromine is even under regulation as it may form
potentially carcinogenic BrO3
-
as a byproduct in drinking water disinfection,
requiring speciation analysis for separating other bromine containing species
such as bromide (Br-
). Bromine has two equally abundant isotopes, which
may only be interfered through polyatomic species in presence of high
concentrations of transition metals such as copper (e.g., 63Cu16O+
and 65Cu16O+
),
or nickel (62Ni16O1
H+
and 64Ni16O1
H+
). In addition, high concentrations of potassium
may lead to the formation of 40Ar39K+
, again interfering with the commonly
analyzed isotope, 79Br. In case the presence of rare earth elements is likely
in a sample, the formation of doubly charged species of gadolinium (158Gd++),
dysprosium (157Dy++ and 158Dy++) and erbium (162Er++) may affect the detection of
bromine. Because of the higher abundance of gadolinium among all rare earth
elements, 79Br is again likely more affected. However, because of its reactivity
towards oxygen, all of the aforementioned interferences are safely removed
using oxygen and a mass shift reaction. Bromine itself has the potential to
interfere on 80Se and 82Se through the formation of hydrides (79Br1
H+
and 81Br1
H+
,
respectively).
Element Bromine
Available isotopes 79Br (50.69%), 81Br (49.31%)
1st Ionization Potential 11.81 eV
Q1 resolution Intelligent Mass Selection (iMS)
Preferred analysis modes
Reaction Finder Default Alternatives
TQ-O2
(79Br16O) SQ-KED, TQ-NH3
(79Br)
23 Back to Periodic Table
Strontium
Yttrium
As with the majority of the alkaline earth elements, strontium forms a
considerable amount of doubly charged ions and well as singly charged ions,
leading to an interference on 44Ca (through 88Sr++). Out of its four isotopes, 88Sr is
usually applied for analysis. In geochemical analysis, the isotope ratio of 88Sr/87Sr
is of interest for age determinations. However, 87Rb creates an isobaric overlap,
which can be removed through a mass shift reaction (forming 87Sr16O+
) using O2
.
Other gases, such as N2O or SF6
have been described for this application as
well, but are of limited use in a multi-element analysis.
Yttrium is, like scandium or germanium, an element often recommended as an
internal standard option in many regulatory methods. Typically, the formation of
polyatomic interferences on its only isotope, 89Y is rare, so that even standard
mode (no interference removal) allows interference free detection.
Element Strontium
Available isotopes 84Sr (0.56%), 86Sr (9.86%), 87Sr (7.00%), 88Sr (82.58%)
Ionization Potential 5.70 eV (11.03 eV)
Q1 resolution Intelligent Mass Selection (iMS)
Preferred analysis modes
Reaction Finder Default Alternatives
TQ-O2
(88Sr16O) SQ-KED
Element Yttrium
Available isotopes 89Y (100%)
Ionization Potential 6.22 eV (12.23 eV)
Q1 resolution Intelligent Mass Selection (iMS)
Preferred analysis modes
Reaction Finder Default Alternatives
TQ-O2
(89Y16O) SQ-KED, TQ-NH3
(89Y)
Rubidium
Rubidium is a typical alkali metal with low first ionization potential, but very high
second ionization potential. None of its two isotopes is significantly interfered
by polyatomic species, but its major isotope, 85Rb, can be slightly positively
biased when using scandium as an internal standard (formation of 40Ar45Sc+
). The
low reactivity of rubidium to O2
(similar for all the alkali metals) can be used to
resolve the potential isobaric overlap with 87Sr, by enabling 87Sr to be converted
to SrO+
to move it away from 87Rb.
Element Rubidium
Available isotopes 85Rb (72.17%), 87Rb (27.84%)
Ionization Potential 4.18 eV
Q1 resolution Intelligent Mass Selection (iMS)
Preferred analysis modes
Reaction Finder Default Alternatives
SQ-KED TQ-O2
(85Rb),
TQ-NH3
(85Rb)
24 Back to Periodic Table
Zirconium
Niobium
The main isotope, 90Zr, makes up for more than half of all atoms of the element
found in nature. Due to its unique properties, it is often applied in alloys and
advanced materials, hence it will often be analyzed in combination with other
metals, which may be interfered by its presence (e.g., palladium or silver).
Zirconium is very reactive with O2
, and readily forms higher oxides (such as
ZrO2
+
, ZrO3
+
) as well as ZrO+
in the collision cell. This property is useful for
removing ZrO+
and ZrOH+
interferences on Pd, Ag and Cd, which are not
reactive towards oxygen.
Niobium is monoisotopic at m/z 93. There are little polyatomic interferences
to be expected under normal conditions, for example hydrides of zirconium or
molybdenum (92Zr1
H+
and 92Mo1
H+
), but also species of selenium formed in the
plasma (e.g., 77Se16O+
).
An overlap by doubly charged ions of tungsten, rhenium or osmium might
be possible, but is unlikely based on the 2nd ionization potentials of these
elements, all exceeding 16 eV (and therefore the ionization potential of argon)
significantly. Niobium reacts with oxygen in a mass shift reaction and can
therefore be easily analyzed free from interferences using a triple quadrupole
ICP-MS. It forms also some product ions with ammonia, but the on mass
reaction is typically providing a better sensitivity.
Element Zirconium
Available isotopes
90Zr (51.45%), 91Zr (11.22%), 92Zr (17.15%), 94Zr (17.38%), 96Zr (2.80%)
Ionization Potential 6.63 eV (13.16 eV)
Q1 resolution Intelligent Mass Selection (iMS)
Preferred analysis modes
Reaction Finder Default Alternatives
TQ-O2
(90Zr16O) SQ-KED, TQ-NH3
(90Zr)
Element Niobium
Available isotopes 93Nb (100%)
1st Ionization Potential 6.76 eV
Q1 resolution Intelligent Mass Selection (iMS)
Preferred analysis modes
Reaction Finder Default Alternatives
TQ-O2
(93Nb16O) SQ-KED, TQ-NH3
(93Nb)
25 Back to Periodic Table
Molybdenum
Molybdenum has a number of stable isotopes between m/z 92 and m/z 100.
Although potentially interfered by several oxides, nitrides or argides formed
by other elements, all of these interferences can be efficiently reduced using
KED. It is reactive with oxygen and can be analyzed via a mass shift process.
This is used to remove molybdenum based interferences on cadmium. Similar
to zirconium, molybdenum is also very reactive to oxygen and forming higher
oxides.
Element Molybdenum
Available isotopes
92Mo (14.84%), 94Mo (9.25%), 95Mo (15.92%), 96Mo (16.68%), 97Mo (9.55%), 98Mo (24.13%), 100Mo (9.63%)
Ionization Potential 7.09 eV
Q1 resolution Intelligent Mass Selection (iMS)
Preferred analysis modes
Reaction Finder Default Alternatives
TQ-O2
(98Mo16O) SQ-KED
Silver
Silver is increasingly being used in the production of nanomaterials with
antibacterial properties in a variety of consumer products. This is leading
to an increase of the amount of silver found in the environment, mostly in
its ionic form Ag+
, but also as nanomaterial. Although its two isotopes are
almost equally abundant, 107Ag is the preferred isotope for analysis. Silver is
potentially interfered when metals such as zirconium or niobium are present.
These elements form oxide (93Nb16O interfering on 109Ag) and hydroxide ions
(
90Zr16O1
H interfering on 107Ag), which can be efficiently removed using O2
. Silver
is not reactive with O2
due to its noble chemical nature, and so the above
interferences can be further oxidized and hence mass separated.
Element Silver
Available isotopes 107Ag (51.84%), 109Ag (48.16%)
Ionization Potential 7.58 eV
Q1 resolution Intelligent Mass Selection (iMS)
Preferred analysis modes
Reaction Finder Default Alternatives
SQ-KED TQ-O2
(107Ag)
26 Back to Periodic Table
Cadmium
Cadmium has a total of eight stable isotopes. Although 114Cd has the highest
abundance, the preferred choice in ICP-MS is usually 111Cd due to the absence
of isobaric overlaps with isotopes of palladium or tin, which affect all other
isotopes of Cd. The most prominent interference on 111Cd is caused through
95Mo16O+
, but also zirconium can interfere (94Zr16O1
H+
). As cadmium is not reactive
towards O2
, an oxidation reaction of molybdenum (forming predominantly
95Mo16O2
+
) can be used in an on mass measurement.
Element Cadmium
Available isotopes
106Cd (1.25%), 108Cd (0.89%), 110Cd (12.49%), 111Cd (12.80%), 112Cd (24.13%), 113Cd (12.22%), 114Cd (28.73%), 116Cd (12.49%)
Ionization Potential 8.99 eV
Q1 resolution Intelligent Mass Selection (iMS)
Preferred analysis modes
Reaction Finder Default Alternatives
SQ-KED TQ-O2
(111Cd)
Indium
Indium is the only element in the Periodic Table that has no isotope free
from isobaric overlap. Both isotopes, 113In and 115In, are interfered through
other isotopes of either cadmium or tin. However, the abundance of 113In is
significantly lower compared to 115In, so that only the latter isotope is used for
analysis. Indium is commonly used as an internal standard, but mathematical
correction for Cd or Sn interference is feasible to avoid negative impact on the
results, since polyatomic interferences or contributions of doubly charged ions
are unlikely. Indium shows no significant reactivity to O2
, NH3
or H2
, so that all
measurement modes will be on mass.
Element Indium
Available isotopes 113In (4.30%), 115In (95.70%)
Ionization Potential 5.79 eV
Q1 resolution Intelligent Mass Selection (iMS)
Preferred analysis modes
Reaction Finder Default Alternatives
SQ-KED TQ-O2
(115In),
TQ-NH3
(115In)
27 Back to Periodic Table
Tin
Tin is the element with the highest number of stable isotopes found in the
Periodic Table with a total of 10 isotopes. Tin therefore causes a significant
number of isobaric overlaps, which can in most cases be accounted for by
using mathematical corrections. For ICP-MS analysis, there are two isotopes
which are recommended for analysis, 118Sn and 120Sn, as these are the most
abundant isotopes. Both are free from isobaric overlaps through other elements
and little affected by polyatomic interferences in the most typical sample
matrices. Tin is commonly analyzed using kinetic energy discrimination. It
shows no significant reactivity towards the commonly used reactive gases.
Element Tin
Available isotopes
112Sn (0.97%), 114Sn (0.65%), 115Sn (0.36%), 116Sn (14.53%), 117Sn (7.68%), 118Sn (24.22%), 119Sn (8.58%), 120Sn (32.59%), 122Sn (4.63%), 124Sn (5.79%)
Ionization Potential 7.34 eV (14.63 eV)
Q1 resolution Intelligent Mass Selection (iMS)
Preferred analysis modes
Reaction Finder Default Alternatives
SQ-KED TQ-NH3
(118, 120Sn)
Antimony
Antimony has two isotopes with similar abundance, and both are equally
suitable for ICP-MS analysis. The use of kinetic energy discrimination is mostly
sufficient for analysis, as polyatomic interferences will only be formed in the
presence of certain elements, such as silver or palladium (as oxides or nitrides).
Like tin, antimony does not show reactivity to either O2
, H2
, or NH3.
Element Antimony
Available isotopes 121Sb (57.30%), 123Sb (42.70%)
Ionization Potential 8.64 eV
Q1 resolution Intelligent Mass Selection (iMS)
Preferred analysis modes
Reaction Finder Default Alternatives
SQ-KED TQ-NH3
(121Sb)
28 Back to Periodic Table
Tellurium
Tellurium is an element occasionally used as an internal standard, but is also
becoming more important for analysis in environmental studies in order to
better understand its toxicity and pathways as a contaminant. As a non-metal,
it´s ionization potential is high, limiting the ion yield, and its most abundant
isotopes are interfered by isobaric overlaps of xenon (128Xe on 128Te, having an
abundance of 31.69%), and more importantly barium on the most abundant
isotope 130Te (with an abundance of 33.80%). However, using O2
as a reactive
gas, xenon ions are efficiently removed from the ion beam, whereas barium
ions undergo an oxidation and are hence separated in the analyzing quadrupole
after reaction. Tellurium can therefore be detected interference free using the
most abundant isotope with triple quadrupole ICP-MS, whereas less abundant
125Te would be the common choice on a single quadrupole instrument.
Element Tellurium
Available isotopes
120Te (0.10%), 122Te (2.60%), 123Te (0.91%), 124Te (4.82%), 125Te (7.14%), 126Te (18.95%), 128Te (31.69%), 130Te (33.80%)
Ionization Potential 9.01 eV
Q1 resolution Intelligent Mass Selection (iMS)
Preferred analysis modes
Reaction Finder Default Alternatives
SQ-KED TQ-O2
(130Te)
Iodine
Iodine is monoisotopic in nature (127I), but has a few artificial isotopes, such as
129I or 131I, which are of analytical interest. These isotopes are long-lived therefore
subject to environmental forensic analysis. Due to the presence of Xe as a
common impurity in argon gas, there is an isobaric interference on both 129I
and 131I, which can be removed using O2
. Moreover, if trace analysis of 129I next
to high amounts of natural iodine is required, significant backgrounds can
be formed through the formation of 127I
1
H2
, which can be reduced using triple
quadrupole instrumentation.
Element Iodine
Available isotopes 127I (100%), artificial isotopes 129I and 131I
Ionization Potential 10.45 eV
Q1 resolution Intelligent Mass Selection (iMS)
Preferred analysis modes
Reaction Finder Default Alternatives
SQ-KED TQ-O2
(127I)
29 Back to Periodic Table
Cesium
Cesium is monoisotopic at m/z 133, but has long-lived artificial isotopes, such
as 137Cs, which are of interest in environmental forensics. Whereas 133Cs shows
no significant interferences, 137Cs is interfered through an isobaric overlap with
137Ba. In order to separate the elements from each other, O2
can be applied,
leading to a mass shift reaction of Ba and hence interference removal.
Element Cesium
Available isotopes 133Cs (100%), artificial isotope 137Cs
Ionization Potential 3.89 eV
Q1 resolution Intelligent Mass Selection (iMS)
Preferred analysis modes
Reaction Finder Default Alternatives
SQ-KED TQ-O2
(133Cs)
Barium
Barium is abundant in nature and has a total of 7 isotopes. For the quantitative
analysis of barium either 137Ba or 138Ba are applied (using SQ-KED or TQ-O2
mode),
but the other minor isotopes need to be considered as potential interferences
on other elements, such as tellurium or cesium. Due to its low second ionization
potential, barium is a likely interference on elements such as gallium through the
formation of doubly charged ions.
Element Barium
Available isotopes
130Ba (0.11%), 132Ba (0.10%), 134Ba (2.42%), 135Ba (6.59%), 136Ba (7.85%), 137Ba (11.23%), 138Ba (71.70%)
Ionization Potential 5.21 eV (10.00 eV)
Q1 resolution Intelligent Mass Selection (iMS)
Preferred analysis modes
Reaction Finder Default Alternatives
SQ-KED TQ-O2
(138Ba16O)
30 Back to Periodic Table
Element Lanthanum
Available isotopes 138La (0.09%), 139La (99.91%)
Ionization Potential 5.58 eV (11.06 eV)
Q1 resolution Intelligent Mass Selection (iMS)
Preferred analysis modes
Reaction Finder Default Alternatives
SQ-KED TQ-O2
(139La16O)
Element Cerium
Available isotopes 136Ce (0.19%), 138Ce (0.25%), 140Ce (88.48%), 142Ce (11.08%)
Ionization Potential 5.54 eV (10.88 eV)
Q1 resolution Intelligent Mass Selection (iMS)
Preferred analysis modes
Reaction Finder Default Alternatives
TQ-O2
(140Ce16O) SQ-KED
Element Praseodymium
Available isotopes 141Pr (100%)
Ionization Potential 5.46 eV (10.57 eV)
Q1 resolution Intelligent Mass Selection (iMS)
Preferred analysis modes
Reaction Finder Default Alternatives
TQ-O2
(141Pr16O) SQ-KED
Additional element descriptions follow on next page.
The rare earth elements comprise a group of 14 elements (from lanthanum
to lutetium) in the mass range between 139 and 176. Because of their high
chemical similarity, these elements often occur in combination with each
other, the most abundant single element out of the series being gadolinium.
An exception is promethium, which does not occur naturally and is not
included here. All rare earth elements are characterized by a low 1st ionization
potential (yielding a high detection sensitivity at normally low backgrounds),
but also a moderate 2nd ionization potential, leading to a variety of potentially
occurring doubly charged interferences. Furthermore, all rare elements tend
to form stable oxides, so that although there is an isotope free from isobaric
overlaps for each element, these polyatomic interferences can be of concern
when measuring heavier rare earth elements in presence of their lower mass
homologues. This is especially obvious for the determination of ytterbium in the
presence of gadolinium. Both elements have an identical number of isotopes
with almost identical abundances, but exactly 16 mass units apart. Therefore,
the presence of gadolinium makes the determination of ytterbium at low levels
very difficult. However, these elements also show different reactivities towards
NH3, so that resolution of the aforementioned interferences is possible using
triple quadrupole ICP-MS. Elements such as Pr, Eu, Dy, Ho, Er, Tm and Yb do
not (or only to a negligible extent) react with NH3, but oxide interferences are
effectively removed. The remaining elements, La, Ce, Nd, Sm, Gd, Tb and Lu
do react with NH3, but less effectively compared to the reaction with O2
. Often,
a reaction with O2
(and hence the formation of the MO+
product ion) is the
preferred choice. Element descriptions follow on next pages.
Rare Earth Elements
31 Back to Periodic Table
Rare Earth Elements continued
Element Gadolinium
Available isotopes
152Gd (0.20%), 154Gd (2.18%), 155Gd (14.80%), 156Gd (20.47%), 157Gd (15.65%), 158Gd (24.84%), 160Gd (21.86%)
Ionization Potential 6.15 eV (12.13 eV)
Q1 resolution Intelligent Mass Selection (iMS)
Preferred analysis modes
Reaction Finder Default Alternatives
TQ-O2
(157Gd16O) SQ-KED
Element Terbium
Available isotopes 159Tb (100%)
Ionization Potential 5.86 eV (11.50 eV)
Q1 resolution Intelligent Mass Selection (iMS)
Preferred analysis modes
Reaction Finder Default Alternatives
TQ-O2
(159Tb16O) SQ-KED
Element Dysprosium
Available isotopes
156Dy (0.06%), 158Dy (0.10%), 160Dy (2.34%), 161Dy (18.90%), 162Dy (25.50%), 163Dy (24.90%), 164Dy (28.20%)
Ionization Potential 5.94 eV (11.71 eV)
Q1 resolution Intelligent Mass Selection (iMS)
Preferred analysis modes
Reaction Finder Default Alternatives
TQ-O2
(163Dy16O) SQ-KED
Element Neodymium
Available isotopes
142Nd (27.13%), 143Nd (12.18%), 144Nd (23.80%), 145Nd (8.30%), 146Nd (17.19%), 148Nd (5.76%), 150Nd (5.64%)
Ionization Potential 5.53 eV (10.78 eV)
Q1 resolution Intelligent Mass Selection (iMS)
Preferred analysis modes
Reaction Finder Default Alternatives
TQ-O2
(144Nd16O) SQ-KED
Element Samarium
Available isotopes
144Sm (3.10%), 147Sm (15.00%), 148Sm (11.30%), 149Sm (13.80%), 150Sm (7.40%), 152Sm (26.70%), 154Sm (22.70%)
Ionization Potential 5.64 eV (11.09 eV)
Q1 resolution Intelligent Mass Selection (iMS)
Preferred analysis modes
Reaction Finder Default Alternatives
TQ-O2
(149Sm16O) SQ-KED
Element Europium
Available isotopes 151Eu (47.80%), 153Eu (52.20%)
Ionization Potential 5.67 eV (11.25 eV)
Q1 resolution Intelligent Mass Selection (iMS)
Preferred analysis modes
Reaction Finder Default Alternatives
SQ-KED TQ-O2
(153Eu)
Additional element descriptions follow on next page.
32 Back to Periodic Table
Element Holmium
Available isotopes 165Ho (100%)
Ionization Potential 6.02 eV (11.82 eV)
Q1 resolution Intelligent Mass Selection (iMS)
Preferred analysis modes
Reaction Finder Default Alternatives
TQ-O2
(165Ho16O) SQ-KED
Element Erbium
Available isotopes
162Er (0.14%), 164Er (1.61%), 166Er (33.60%), 167Er (22.95%), 168Er (26.80%), 170Er (14.90%)
Ionization Potential 6.10 eV (11.92 eV)
Q1 resolution Intelligent Mass Selection (iMS)
Preferred analysis modes
Reaction Finder Default Alternatives
TQ-O2
(166Er16O) SQ-KED
Element Tullium
Available isotopes 169Tm (100%)
Ionization Potential 6.18 eV (12.02 eV)
Q1 resolution Intelligent Mass Selection (iMS)
Preferred analysis modes
Reaction Finder Default Alternatives
TQ-O2
(169Tm16O) SQ-KED
Rare Earth Elements continued
Element Ytterbium
Available isotopes
168Yb (0.13%), 170Yb (3.05%), 171Yb (14.30%), 172Yb (21.90%), 173Yb (16.12%), 174Yb (31.80%), 176Yb (12.70%)
Ionization Potential 6.25 eV (12.18 eV)
Q1 resolution Intelligent Mass Selection (iMS)
Preferred analysis modes
Reaction Finder Default Alternatives
SQ-KED TQ-O2
(172Yb)
Element Lutetium
Available isotopes 175Lu (97.41%), 176Lu (2.59%)
Ionization Potential 5.43 eV (13.89 eV)
Q1 resolution Intelligent Mass Selection (iMS)
Preferred analysis modes
Reaction Finder Default Alternatives
TQ-O2
(175Lu16O) SQ-KED
33 Back to Periodic Table
Hafnium
Tantalum
Hafnium is rarely of interest for quantitation, however, Hf isotopes can be
relevant in geosciences for the determination of the petrogenesis of igneous
rocks, and can be used to show crust or mantle mixing and weathering cycles.
However, there are isobaric overlaps caused by ytterbium and tungsten
on some of the key isotopes, which can be removed using NH3. Whereas
ytterbium is close to unreactive to this gas, a variety of cluster ions are formed
with hafnium, mostly following the general formula [Hf(NH2
)(NH3)x], the most
abundant one being [Hf(NH2
)(NH3)
3]
+
. Other rare earth elements, potentially more
reactive towards NH3, are efficiently removed in the first quadrupole.
For quantitative analysis, the selection of TQ-O2
mode using 178Hf is a viable way
of assuring full interference removal and increasing the detection sensitivity.
Tantalum is quasi-monoisotopic, as its major isotope, 181Ta, has an abundance
of 99.99%. Tantalum is one of the rarest elements on earth, but is used in
a variety of applications, from electronic components to medical implants.
Therefore, it is not commonly analyzed. The only relevant polyatomic
interferences can be formed with rare earth elements, for example 165Ho16O+
,
if Ho is present in the sample. However, Ta reacts efficiently with oxygen, so
that formation of 181Ta16O is a good way of eliminating the aforementioned Ho
interference.
Element Hafnium
Available isotopes
174Hf (0.16%), 176Hf (5.21%), 177Hf (18.61%), 178Hf (27.30%), 179Hf (13.63%), 180Hf (35.10%)
Ionization Potential 6.83 eV (14.93 eV)
Q1 resolution Intelligent Mass Selection (iMS)
Preferred analysis modes
Reaction Finder Default Alternatives
TQ-O2
(178Hf16O) TQ-NH3
(178[NH(NH2
)(NH3
)
3
)]
Element Tantalum
Available isotopes 180Ta (0.01%), 181Ta (99.99%)
Ionization Potential 7.89 eV
Q1 resolution Intelligent Mass Selection (iMS)
Preferred analysis modes
Reaction Finder Default Alternatives
TQ-O2
(181Ta16O) SQ-KED, TQ-NH3
(181Ta)
34 Back to Periodic Table
Tungsten
Rhenium
Tungsten has a total of five stable isotopes, of which 182W is the most common
choice for analysis. As is generally the case in the high mass range, polyatomic
interferences on W are rare, so that in most cases, only isobaric overlaps are
considered. Some isotopes of tungsten may be affected by oxide ions of less
abundant rare earth elements, such as thulium or erbium. Tungsten itself may
be found as an interference on mercury, especially through its major isotopes
184W and 186W (interfering as WO+
or WOH+
ions).
Rhenium is occasionally used as an internal standard due its rare occurrence
in nature, mostly using its minor isotope 185Re, which is not affected by
isobaric overlaps.
Element Tungsten
Available isotopes
180W (0.13%), 182W (26.30%), 183W (14.30%), 184W (30.67), 186W (28.60%)
Ionization Potential 7.98 eV
Q1 resolution Intelligent Mass Selection (iMS)
Preferred analysis modes
Reaction Finder Default Alternatives
SQ-KED TQ-NH3
(
265[
182W(14N1
H)(14N1
H3)
4)]
Element Rhenium
Available isotopes 185Re (37.40%), 187Re (62.60%)
Ionization Potential 7.88 eV
Q1 resolution Intelligent Mass Selection (iMS)
Preferred analysis modes
Reaction Finder Default Alternatives
SQ-KED TQ-O2
(185Re),
TQ-NH3
(185Re)
35 Back to Periodic Table
Ruthenium
Rhodium
Ruthenium is commonly determined using the third most abundant of its
seven stable isotopes, 101Ru. Other isotopes, such as the most abundant 102Ru
are affected by isobaric overlaps. Typically, there are almost no polyatomic
interferences affecting the detection of 101Ru, so that even standard mode (CRC
used as an ion guide only) would provide accurate results. As with all platinum
group metals, ruthenium is not reactive to O2
, but slightly reactive to NH3,
forming a cluster ion through the addition of one NH3
molecule per atom.
Rhodium is monoisotopic with its only isotope being 103Rh. It is often analyzed
in order to assess purity of metals or metal alloys and can be interfered in the
presence of high concentrations of Cu (63Cu40Ar+
) and lead (206Pb++). Whereas
the copper based interference can be effectively removed using KED, the lead
induced interference can only be removed using O₂ as a reactive gas, in which
case, 103Rh will be determined on mass. Due to the frequent use of rhodium as
an internal standard, interferences caused by higher concentrations of lead can
be misinterpreted as an unexplained matrix effect.
Element Ruthenium
Available isotopes
96Ru (5.52%), 98Ru (1.88%), 99Ru (12.70%), 100Ru (12.60%), 101Ru (17.00%), 102Ru (31.60%), 104Ru (18.70%)
Ionization Potential 7.36 eV
Q1 resolution Intelligent Mass Selection (iMS)
Preferred analysis modes
Reaction Finder Default Alternatives
SQ-KED SQ-N/A
Element Rhodium
Available isotopes 103Rh (100%)
Ionization Potential 7.46 eV
Q1 resolution Intelligent Mass Selection (iMS)
Preferred analysis modes
Reaction Finder Default Alternatives
SQ-KED TQ-O2
(103Rh)
Platinum Group Metals (PGMs)
36 Back to Periodic Table
Palladium
Osmium
Palladium has a series of isotopes, the default selection often being 105Pd due
to the absence of isobaric overlaps (caused by either ruthenium or cadmium).
However, since 89Y is an element often used as an internal standard, there
may be elevated backgrounds or false positive results observed through the
formation of 89Y16O+
. Like most PGMs, Pd is unreactive towards O2
and can be
analyzed interference free on mass.
Osmium has a total of 7 stable isotopes, but despite the high number, 192Os
still shows a reasonable abundance of 41.00%. However, due to isobaric
interferences with 192Pt, the preferred isotope for analysis is often 189Os. Due to
a similar reactivity of Pt and Os towards both O2
and NH3, the selection of this
alternative isotope is the best option. Osmium is well known to be affected by
physical interferences and losses occurring during sample preparation and
analysis, especially because of its high volatility in its oxidized form (e.g., after
a microwave digestion). As well as potentially being lost to the atmosphere on
opening the digestion vessel, Os can also be volatilized preferentially in the
spray chamber, which may lead to an overestimation when not calibrated using
standards containing the same chemical form of Os.
Element Palladium
Available isotopes
102Pd (1.02%), 104Pd (11.14%), 105Pd (22.33%), 106Pd (27.33%), 108Pd (26.46%), 110Pd (11.72%)
Ionization Potential 8.34 eV
Q1 resolution Intelligent Mass Selection (iMS)
Preferred analysis modes
Reaction Finder Default Alternatives
SQ-KED TQ-O2
(105Pd)
Element Osmium
Available isotopes
184Os (0.02%), 186Os (1.58%), 187Os (1.60%), 188Os (13.30%), 189Os (16.10%), 190Os (26.40%), 192Os (41.00%)
Ionization Potential 8.71 eV
Q1 resolution Intelligent Mass Selection (iMS)
Preferred analysis modes
Reaction Finder Default Alternatives
SQ-KED TQ-O2
(189Os),
TQ-NH3
(204[
189Os(14N1
H)])
Platinum Group Metals (PGMs)
37 Back to Periodic Table
Iridium
Platinum
Like all platinum group metals, iridium is analyzed as part of the composition
and impurity analysis of ores and refined metals/alloy products. Iridium is also
often used as a high mass internal standard. Its more abundant mass 193
isotope (193Ir) may be interfered by oxide or hydroxide ions of elements such as
lutetium and hafnium, but by itself it can create an oxide interference on 209Bi.
Similar to the aforementioned elements, interferences can be removed through
the use of O2
, to which Ir shows only limited reactivity.
The two most abundant isotopes of platinum are 194Pt and 195Pt (with 32.90
and 33.80% relative abundance), however, both may be interfered through
hafnium oxide and hydroxide interferences. On mass measurements using O2
cell gas are an effective way to remove these interferences. In contrast to other
platinum group metals, platinum also forms product ions with NH3, such as
229[
195Pt(NH3)
2
]
+
.
Element Iridium
Available isotopes 191Ir (37.30%), 193Ir (62.30%)
Ionization Potential 9.12 eV
Q1 resolution Intelligent Mass Selection (iMS)
Preferred analysis modes
Reaction Finder Default Alternatives
SQ-KED TQ-O2
(193Ir)
Element Platinum
Available isotopes
190Pt (0.01%), 192Pt (0.79%), 194Pt (32.90%), 195Pt (33.80%), 196Pt (25.30%), 198Pt (7.20%)
Ionization Potential 9.02 eV
Q1 resolution Intelligent Mass Selection (iMS)
Preferred analysis modes
Reaction Finder Default Alternatives
SQ-KED TQ-O2
(195Pt) or
TQ-NH3
(229[
195Pt(NH3)
2
])
Platinum Group Metals (PGMs)
38 Back to Periodic Table
Gold
Mercury
Gold is often used for stabilization and reduction of memory effects of mercury,
commonly during the analysis of environmental samples (drinking waters or
waste waters), food samples or pharmaceutical products. In recent years, it
has started to be used in nanomaterial production and can therefore also be
found increasingly in the environment. Gold is an important analyte for the
assessment of the content or impurity levels in ores and metals/alloys, where
its only isotope 197Au can be interfered by 181Ta16O+
. The determination of gold
in materials containing platinum may be affected through hydride formation
and peak tailing of the 196Pt and 198Pt isotopes, which can be reduced through
the use O2
in the collision cell in combination with high mass resolution on the
analyzing quadrupole. Like platinum, cluster ions of identical composition can
be formed with NH3
as a reactive gas.
Mercury is a key element in many regulated methods due to its elevated
toxicity. Out of its seven stable isotopes, commonly the most abundant 202Hg is
applied for analysis. Occasionally, interferences caused through the presence
of tungsten (forming for example 186W16O+
) can be found to bias results. Similar
to the removal of molybdenum interferences on cadmium, O2
can be used to
convert WO+
species into WO2
+
derivatives, whereas mercury is not reactive and
can be determined interference free on mass.
Element Gold
Available isotopes 197Au (100%)
Ionization Potential 9.23 eV
Q1 resolution Intelligent Mass Selection (iMS)
Preferred analysis modes
Reaction Finder Default Alternatives
SQ-KED TQ-O2
(197Au) or
TQ-NH3
(231[
197Au(NH3)
2
])
Element Mercury
Available isotopes
196Hg (0.14%), 198Hg (10.02%), 199Hg (16.84%), 200Hg (23.13%), 201Hg (13.22%), 202Hg (29.80%), 204Hg (6.85%)
Ionization Potential 10.44 eV
Q1 resolution Intelligent Mass Selection (iMS)
Preferred analysis modes
Reaction Finder Default Alternatives
SQ-KED TQ-O2
(202Hg)
39 Back to Periodic Table
Thallium
Lead
Thallium is a highly toxic metal, having two isotopes, which can normally
considered interference free. It shows limited reactivity to both O2
and NH3, but
formation of product ions is less favorable compared to measuring unreacted Tl.
Lead is an exception in the Periodic Table as its isotopic composition may vary
considerably in different regions. This is because three out of the four stable
Pb isotopes are formed through radioactive decay of uranium or thorium. It
is common practice to calculate the sum of all isotopes rather than using the
result of a single isotope for quantitation. The use of KED mode is the method
of choice, as polyatomic interferences are seldomly observed for lead. An
exception is the determination of lead isotope ratios, which is of high interest
in geosciences. The variable formation of 206Pb, 207Pb and 208Pb is a wellaccepted way to determine the age of a rock. However, the 204Pb isotope (the
only one not formed through radioactive decay) has an isobaric interference
from 204Hg. Whereas a single quadrupole ICP-MS would have to rely on
mathematical correction, triple quadrupole ICP-MS systems can fully eliminate
the interference using NH3. Whereas lead is not reactive to NH3, Hg is efficiently
removed.
Element Thallium
Available isotopes 203Tl (29.52%), 205Tl (70.48%)
Ionization Potential 6.11 eV
Q1 resolution Intelligent Mass Selection (iMS)
Preferred analysis modes
Reaction Finder Default Alternatives
SQ-KED TQ-O2
, TQ-NH3
(205Tl)
Element Lead
Available isotopes
204Pb (1.40%), 206Pb (24.10%), 207Pb (22.10%), 208Pb (52.40%)
Ionization Potential 7.42 eV (15.03 eV)
Q1 resolution Intelligent Mass Selection (iMS)
Preferred analysis modes
Reaction Finder Default Alternatives
SQ-KED TQ-NH3
(204-208Pb)
40 Back to Periodic Table
Bismuth
Thorium, Uranium
Bismuth is monoisotopic and often used as an internal standard for heavy
elements such as lead or mercury. It is normally not interfered and unreactive to
the common gases O2
, NH3
and H2
. However, it can be present as an impurity
in some samples.
Both these elements are only still present in nature because of the extremely
long half-lives of some of their isotopes. Other isotopes may exist as decay
products, but are often short lived and hence not relevant for quantitative
assessment. For thorium, the 232Th isotope is the most stable, having a halflife of around 14 billion years. It is essentially monoisotopic in practice, but
detectable levels of 230Th (with a half-life of 75 thousand years) have been found
in deep ocean waters and sediments. For uranium the 238U isotope (with a
half-life of 4.5 billion years) has the highest mass and also the highest natural
abundance of all U isotopes. The second most abundant isotope, 235U, has a
half-life of 700 million years. Due to their low ionization potential, both elements
can be measured with high ion yield and hence high sensitivity. For uranium,
the use of O2
and subsequent conversion into 238U16O2
+
is an option to improve
sensitivity even further. Uranium can cause peak tailing onto adjacent masses
(especially m/z 237 and 239), but the use of triple quadrupole technology also
allows the impact of this tailing to be significantly reduced by mass filtration in
Q1 and Q3 respectively, as well as by differential reactivity of isotopes such as
237Np and 239Pu towards O2
.
Element Bismuth
Available isotopes 209Bi (100%)
Ionization Potential 7.29 eV
Q1 resolution Intelligent Mass Selection (iMS)
Preferred analysis modes
Reaction Finder Default Alternatives
SQ-KED TQ-O2
, TQ-NH3
(209Bi)
Element Thorium
Available isotopes 232Th (100%), half life 1.405 x 1010 years
1st Ionization Potential 6.08 eV (11.50 eV)
Q1 resolution Intelligent Mass Selection (iMS)
Preferred analysis modes
Reaction Finder Default Alternatives
SQ-KED TQ-O2
(232Th)
Element Uranium
Available isotopes
234U (0.01%), 235U (0.72%), 238U (99.27%)
half live between 2.5 x 105
and 4.5 x 109
years
Ionization Potential 6.19 eV (14.72 eV)
Q1 resolution Intelligent Mass Selection (iMS)
Preferred analysis modes
Reaction Finder Default Alternatives
SQ-KED TQ-O2
(238U16O2
)
Conclusion
The use of triple quadrupole ICP-MS offers new possibilities for the analysis of
elemental contaminants. Whereas for some elements, the default selection of
an analysis mode assuring full interference removal and best limits of detection
is not different between single and triple quadrupole instruments, there are
significant differences for a variety of other elements. In these cases, the use
of triple quadrupole technology allows all types of interferences—polyatomic,
doubly charged ions and isobaric interferences—to be removed. In addition,
the use of reactive gases in combination with suitable bias settings between
the CRC and analyzing quadrupole allows the achievable sensitivity, and hence
the detection limits, to be significantly improved. The use of triple quadrupole
technology therefore allows analysis performance to be enhanced, although
interference removal may not always be an issue for all elements.
At the same time, for a large variety of elements, effective interference removal
can be accomplished through the combination of only two measurement
modes, SQ-KED and TQ-O2
, so that even in high throughout analysis
demanding routine laboratories, the added time per sample will only be in the
order of a few seconds to accommodate the gas exchange between helium
and oxygen. For advanced applications, or special interference challenges,
the use of ammonia can be an attractive alternative, not only to allow highly
selective and specific interference removal, but also to provide a further
improvement of detection sensitivity.
41
© 2020 Thermo Fisher Scientific Inc. All rights reserved. All trademarks are the property of Thermo Fisher Scientific
and its subsidiaries unless otherwise specified. XX44465 0520M
Find out more at thermofisher.com/icp-ms
Our innovative ICP-MS solutions are designed to meet the needs of all
laboratories, from high-throughput and routine to cutting-edge research
facilities. With advanced performance for reliable and accurate data generation
and simple, intuitive workflows for ease-of-use, our ICP-MS instruments
enable you to work smarter now and be ready for future challenges.
Brought to you by
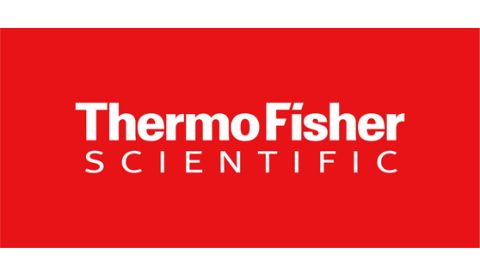
Download Your Guide for FREE Below
Information you provide will be shared with the sponsors for this content.
Technology Networks or its sponsors may contact you to offer you content or products based on your interest in this topic. You may opt-out at any time.
Experiencing issues viewing the form? Click here to access an alternate version