Sensor Multiplexing for Real-Time Decoding of Different Plant Stresses
Distinctive temporal waves of stress molecules could provide early warning system for plant growers.
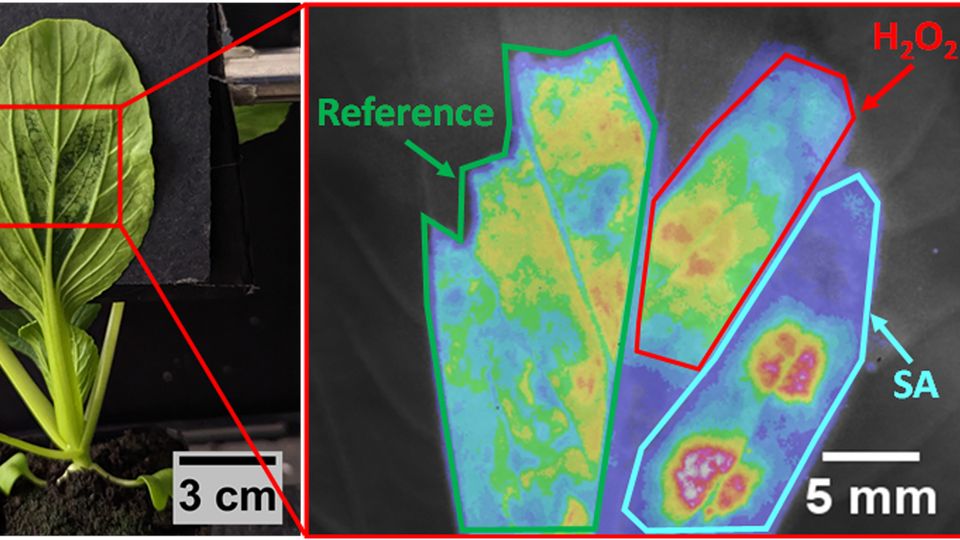
Complete the form below to unlock access to ALL audio articles.
In a multi-institutional study, researchers have developed carbon nanotube sensors to detect the generation of two plant stress signaling molecules: hydrogen peroxide (H2O2) and salicylic acid (SA). These sensors revealed that different stresses produce signaling molecules at different rates and times, creating distinctive, unique temporal waves for each stress type. The work was led by the Disruptive & Sustainable Technologies for Agricultural Precision (DiSTAP) Interdisciplinary Research Group (IRG) of Singapore-MIT Alliance for Research and Technology (SMART), MIT’s research enterprise in Singapore, in collaboration with Temasek Life Sciences Laboratory (TLL) and Massachusetts Institute of Technology (MIT).
Developing novel stress-sensing devices to decode early biochemical signals
Plants experience various types of stress, such as drought, nutrient deficiencies, pest attacks and diseases, and produce a myriad of signaling molecules and hormones to activate defense mechanisms and resilience.
Technologies that enable rapid detection of stress-induced biochemical changes in crops will help farmers enact timely interventions to preserve yield and provide novel insights into the interplay of plant signaling pathways, counteracting stresses and fostering the development of climate-resilient crops.
Our research aimed to develop a novel sensor technology to decode early biochemical changes in plants under stress, enabling early asymptomatic detection. Current techniques for diagnosing plant stress often rely on laboratory analysis, which is time-consuming, expensive and disruptive to plant growth. By combining multiple sensors, we can differentiate stress types based on the timing and rate of production of specific molecules.
Simultaneous monitoring using CoPhMoRe and H2O2 sensing
Our study focused on engineering nano-sensors using carbon nanotubes wrapped in specific polymers. These polymer coatings allow sensors to recognize and bind to specific stress hormones. We created a highly selective sensor that quenches its near-infrared fluorescence upon binding to SA while showing minimal response to other plant hormones. This sensing platform was pioneered at MIT and is known as corona phase molecular recognition (CoPhMoRe). To demonstrate stress differentiation, we paired the SA sensor with an H2O2 sensor. We then simultaneously monitored SA and H2O2 levels in living pak choi (commonly known as Chinese cabbage) plants exposed to light, heat, pathogens and wounding (mimicking insect bites). Each stress triggered a distinct pattern of SA and H2O2 production shortly after the application of stress.
The key findings of the study were that (Figure 1):
- We created the first plant nano-sensor that detects SA, a key stress hormone, directly within living plants.
- By combining our SA sensor with an H2O2 sensor, we observed unique patterns of these molecules produced by plants under different stress conditions.
- The experimental insights from sensor multiplexing have also allowed us to formulate a biochemical kinetic model that effectively captures the temporal features of each stress-specific H2O2 and SA wave.
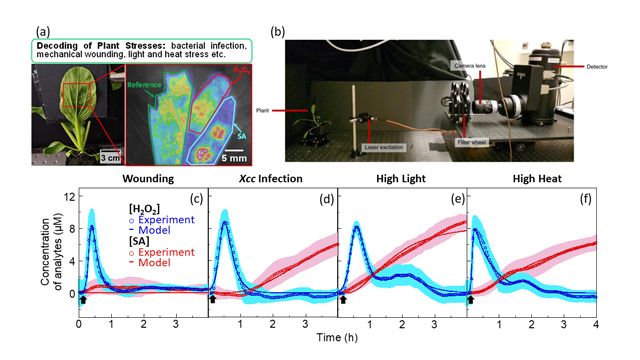
Figure 1: Distinct stress-induced H2O2 and SA waves in living plants subjected to different stresses. (a) Schematic demonstrating the multiplexed sensor platform for the early decoding of different plant stress using an in-plant SA sensor (blue) paired with an H2O2 sensor (red) and a reference sensor (green). (b) Photograph of the experimental setup for the in-plant standoff near-infrared detection. Time-plots of H2O2 concentration derived from nanosensor experiments (blue circles) and mathematical modeling (blue lines), as well as SA concentration derived from nanosensor experiments (red circles) and mathematical modeling (red lines), for pak choi plants that are (c) mechanically-wounded, (d) bacteria-infected, (e) light stressed and (f) heat stressed. Credit: SMART DiSTAP.
Understanding the interplay between H2O2 and SA molecules
Using a novel nanosensor that detects SA selectively, our study has revealed, for the first time, the dynamic interplay between H2O2 and SA molecules during various plant stresses in real time. H2O2 production is observed within minutes, reaching maximum levels and returning back to normal within an hour. Heat, light and bacterial infection triggered SA production within two hours of stress at distinct time points, while mechanical wounding does not stimulate SA production within four hours of stress. These experimental insights allowed us to develop a biochemical kinetic model that captures the unique temporal patterns of H2O2 production for each stress type, forming the basis of early plan stress decoding. Our findings suggest that the distinct timing and levels of H2O2 and SA productivity could influence the effectiveness of the overall stress response. This unveils a stress-specific signature that enables one to identify the stress before visible symptoms appear. The real-time monitoring of plant health using our sensors translates these early signals into actionable information for farmers to address the various plant stresses effectively, aiding in crop yield preservation.
The CoPhMoRe approach is now expanded in this study for the development of a highly selective sensor for SA through a distinct design, synthesis and testing process. For the scientific and plant research community, it has been a longstanding goal to study the crosstalk between plant hormones and their signaling pathways. However, engineering plants with biosensors is challenging beyond model plants. Our sensors are versatile tools that can be easily introduced into any plant species, allowing simultaneous monitoring of multiple stress signaling molecules and bypassing the bottleneck posed by plant transformation.
While our current sensors focus on SA and H2O2, more sensors are needed for the simultaneous and real-time detection of a wider range of plant hormones and signaling molecules to achieve more accurate stress diagnosis. Additionally, our study was conducted in controlled lab settings, whereas real-world agricultural environments present a more complex interplay of stress factors and fluctuating environmental variables that require further sensor evaluation. Although monitoring internal signaling molecules offers the advantage of immediate stress detection, the manual introduction of sensors into plant tissues limits the scalability and throughput of this approach for large-scale agricultural applications. DiSTAP is addressing this limitation by developing a portable system for automatic sensor injection and imaging in plants and using nanosensors to create internet-connected but biocontained “sentinel plants” that can be randomly deployed in agricultural fields. Strategically placed, these sentinel plants can significantly reduce the scale needed for a statistically representative view of overall plant health in the field.
Further unraveling the language of plant stress sensing through sensor multiplexing
Future work will focus on incorporating more sensors and extended monitoring to unravel the signaling network activated by different plant species, especially under complex multiple-stress conditions that are commonplace in real-world agricultural environments. Further studies will be essential to evaluate the life span, stability and diffusion of sensors within plant cells over longer periods of time. These data will aid in refining our biochemical model, leading to more accurate stress identification in crops.
Reference: Ang MCY, Saju JM, Porter TK, et al. Decoding early stress signaling waves in living plants using nanosensor multiplexing. Nat Commun. 2024;15(1):2943. doi:10.1038/s41467-024-47082-1
About the authors:
Dr. Sarojam Rajani is senior principal investigator at Temasek Life Sciences Laboratory (TLL) and principal investigator at Disruptive & Sustainable Technologies for Agricultural Precision (DiSTAP) Interdisciplinary Research Group (IRG) at Singapore-MIT Alliance for Research and Technology (SMART).
Dr. Ang Chunyi Mervin is principal research scientist at the Disruptive & Sustainable Technologies for Agricultural Precision (DiSTAP) Interdisciplinary Research Group (IRG) at Singapore-MIT Alliance for Research and Technology (SMART).