Mass Spectrometry Imaging in Pharmaceutical Development
Mass spectrometry imaging has existed for decades, but recent advances in MS sensitivity and data analysis mean it’s finally coming of age.
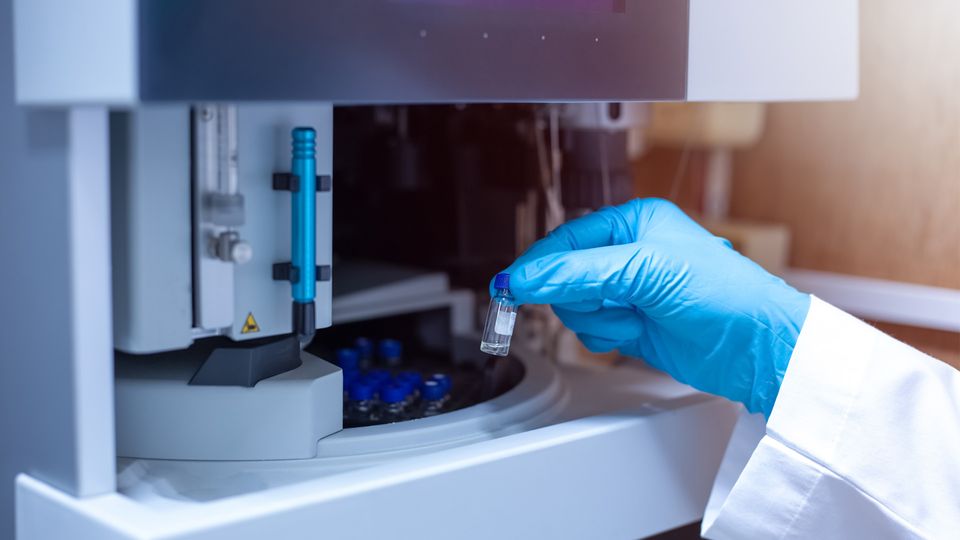
Complete the form below to unlock access to ALL audio articles.
Mass spectrometry imaging (MSI) technology has existed in simple form for decades, but recent advances in MS sensitivity and data analysis means it’s finally coming of age.1 In this article, we explore how MSI is evolving into a high-resolution spatial biology toolset to transform the traditional model of drug discovery and development.
The evolving role of MS in drug development
MS technologies play a crucial role in drug discovery and development, serving as effective tools for the swift identification and quantification of complex molecules.2 Modern MS methods have greatly enhanced the ability to analyze low levels of potential drug molecules – from oligonucleotides, to peptides, proteins and small molecules, and are now used across the drug development pipeline from target discovery, compound screening, and toxicity and quality control testing2, as outlined in Table 1.
Table 1: Applications of mass spectrometry imaging across different stages of R&D.
Stage of drug R&D | Example applications |
Discovery |
|
Development |
|
Quality control |
|
Many of these applications have traditionally involved bulk analysis of cells or tissues, but advances in MSI technologies mean it’s now possible to carry out spatial chemical analysis at the single-cell level. This advancement is paving the way for novel applications in drug discovery and development
What is MS imaging?
“Mass spectrometry imaging is a way to look at metabolism in a spatial manner, which gives you much more information than just analyzing the bulk tissue,” explained Professor Brent Stockwell of Columbia University, USA. “Traditionally, you would administer a drug to an animal, analyze the bulk liver tissue and get a concentration of the drug at a particular timepoint, and this gives you useful information, but this cannot tell you which cells the drug accumulates in and what the impact of the drug was in terms of other metabolites and measures of the cell state.”
MSI achieves this by chemically analyzing snapshots, called a pixel, at a single point within a tissue. For each pixel, molecules are extracted and introduced into the ionization source of the MS instrument, which separates every molecule by its mass-to-charge ratio and determines their relative abundance in the sample. From this, you can visualize the abundance of different molecules across the tissue.
“There’s an inherent trade-off in the MS imaging approach: if you use a bigger pixel size, you cover more tissue but you get less spatial resolution to be able to see how patterns vary,” said Stockwell.
“However, more sensitive MS instruments together with better ionization methods are making it possible to reliably detect minute amounts of sample. This, combined with improved software for data analysis and annotation, is expanding the uses for MS imaging.”
The evolution of MS imaging
MSI was first developed more than 50 years ago using secondary ion mass spectrometry (SIMS), and the most widely used MSI method today is MALDI.1 But despite its widespread use, MALDI-based MSI is not without its limitations – the fragmentation of biological molecules and careful consideration of the matrix for each study are a few examples.1 Other ionization methods have been used for MS imaging, such as desorption electrospray ionization (DESI) and nanoDESI which make it possible to analyze non-volatile molecules without fragmentation.1 Still, it was not until recently that the technology matured to a point that allows for high-impact studies.
“One downside to conventional MALDI methods is the chemical preparation you need to use to be able to detect certain molecules,” explained Professor David Muddiman, of North Carolina State University, USA. Muddiman has spent nearly two decades developing a less destructive ionization approach called matrix-assisted laser desorption electrospray ionization (MALDESI) which makes it possible to directly analyze a diverse range of biological molecules without requiring chemical derivatization in a tissue.1 “For example, neurotransmitters are tiny molecules that the matrix in MALDI interferes with, so you need to treat them with a reagent to bring them into a higher mass range. With MALDESI-based MS imaging, you can analyze the endogenous neurotransmitters in the tissue without any pre-treatment.”
The same applies to glycans – carbohydrate groups added co- and post-translationally to proteins that play crucial roles in biology ‒ which are easily fragmented during conventional MALDI-MS. “If you look in the literature at MS imaging using MALDI, there are very few instances where analysis has been able to identify sialic acids – terminal monosaccharides on carbohydrates – and there’s no method to recover that information through bioinformatics. This is because there’s no way of knowing what the original molecule in the sample was. With our method, we are reading back authentically the biology that’s been presented to us.”
MS imaging opportunities in drug development
The ability to track the single-cell pharmacokinetics and pharmacodynamics of a drug using MS imaging has been coined by Stockwell as “spatial pharmacology”.11 “Spatial biology methods such as spatial transcriptomics or proteomics can give you information about the cell types and cell communities and the state of individual cells,” he explained. “But right now, there’s a layer of information you can’t get directly from those methods – and that’s the small-molecule products of those metabolic reactions. But with MS imaging you can see the abundance of those metabolites across the tissue in a spatially defined manner.”
In the long term, there could be a potential application for MSI in late-stage drug development, where MALDI-based MS is currently the gold standard for characterizing the distribution of drug candidates in development. In the short term, there is a clear role for MSI in the drug discovery pipeline. “I think the demand right now for MS imaging is in looking at disease models versus healthy normal tissues,” noted Stockwell. “It can help to understand disease mechanisms, find new targets and validate drug candidates from screening at an early stage.”
As the technology becomes automated and higher throughput, it becomes a more feasible choice for use in drug screening. “Scientists in biopharma have adapted IR-MALDESI to measure around 22 wells per second for screens involving a simple enzymatic metabolic readout12, and around 1‒3 wells per second for more complex high-content screens,” said Muddiman. “It’s potentially turning the drug discovery pipeline on its head – by starting with the experiment that really matters – the phenotype of a drug candidate, whether it hits its target and identifying any off-target effects.”
Only in the past few years has it become possible to look at single-cell resolution using MSI and it’s still a relatively specialized field. “As companies start to adopt these latest advances that give single-cell resolution, they will gain more useful information and find new applications that will be commonly implemented into their workflows,” said Stockwell.
“The cell is the fundamental unit of biology, it’s the building block of organisms. If you can’t see the individual cells, you’re always going to be somewhat in the dark.”
References
1. Caleb Bagley M, Garrard KP, Muddiman DC. The development and application of matrix assisted laser desorption electrospray ionization: The teenage years. Mass Spectrom Rev. 2023;42(1):35-66. doi: 10.1002/mas.21696
2. Michnowicz J. Mass spectrometry in drug discovery and development. Nat Rev Drug Discov. 2002;1(8):651. doi: 10.1038/nrd886
3. Dueñas ME, Peltier-Heap RE, Leveridge M, Annan RS, Büttner FH, Trost M. Advances in high-throughput mass spectrometry in drug discovery. EMBO Mol Med. 2023;15(1):e14850. doi: 10.15252/emmm.202114850
4. Williams JD, Pu F, Sawicki JW, Elsen NL. Ultra-high-throughput mass spectrometry in drug discovery: fundamentals and recent advances. Expert Opin Drug Discov. 2024;19(3):291-301. doi: 10.1080/17460441.2023.2293153
5. Chu I, Nomeir AA. Utility of mass spectrometry for in-vitro ADME assays. Curr Drug Metab. 2006;7(5):467-477. doi: 10.2174/138920006777697954
6. Ahire D, Kruger L, Sharma S, Mettu VS, Basit A, Prasad B. Quantitative proteomics in translational absorption, distribution, metabolism, and excretion and precision medicine. Pharmacol Rev. 2022;74(3):769-796. doi:10.1124/pharmrev.121.000449
7. Santiago BG, Eisennagel SH, Peckham GE, et al. Perspective on high-throughput bioanalysis to support in vitro assays in early drug discovery. Bioanalysis. 2023;15(3):177-191. doi: 10.4155/bio-2022-0207
8. Robinson MR, Miller RA, Spellman DS. Mass spectrometry-based biomarkers in drug development. Adv Exp Med Biol. 2019;1140:435-449. doi: 10.1007/978-3-030-15950-4_25
9. Deschamps E, Calabrese V, Schmitz I, Hubert-Roux M, Castagnos D, Afonso C. Advances in ultra-high-resolution mass spectrometry for pharmaceutical analysis. Molecules. 2023;28(5):2061. doi: 10.3390/molecules28052061
10. Zhang H, Cui W, Gross ML. Mass spectrometry for the biophysical characterization of therapeutic monoclonal antibodies. FEBS Lett. 2014;588(2):308-317. doi: 10.1016/j.febslet.2013.11.027
11. Rajbhandari P, Neelakantan TV, Hosny N, Stockwell BR. Spatial pharmacology using mass spectrometry imaging. Trends Pharmacol Sci. 2024;45(1):67-80. doi: 10.1016/j.tips.2023.11.003
12. Radosevich AJ, Pu F, Chang-Yen D, et al. Ultra-high-throughput ambient MS: Direct analysis at 22 samples per second by infrared matrix-assisted laser desorption electrospray ionization mass spectrometry. Anal Chem. 2022;94(12):4913-4918. doi: 10.1021/acs.analchem.1c04605