Challenges and Solutions in Cell Therapy Development
As the cell therapy pipeline expands, the alignment of regulatory pathways will be crucial to facilitate access to new treatments for patients worldwide.
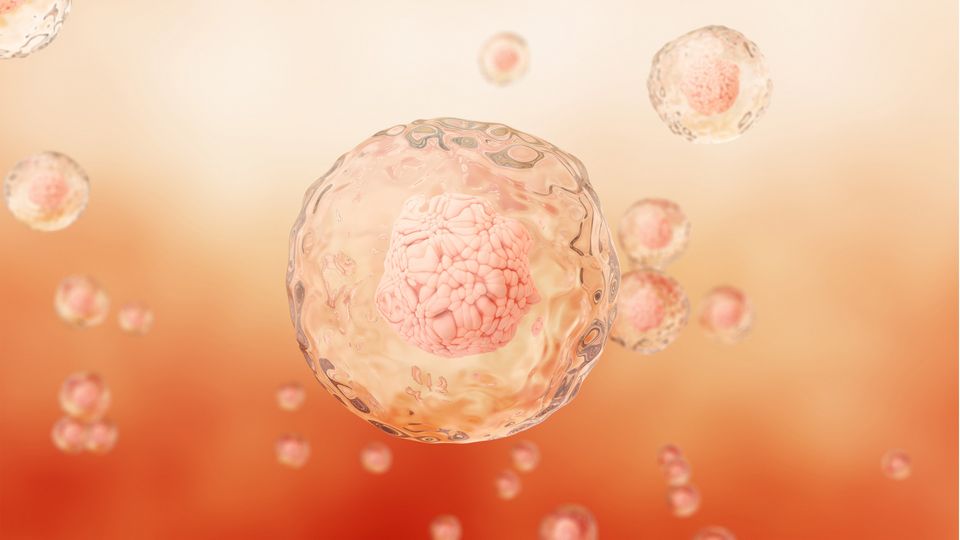
Complete the form below to unlock access to ALL audio articles.
Cell therapy involves the transplantation of live human cells into a patient to repair damaged tissue or cure disease. A variety of cell types either belonging to the patient, in the case of autologous therapies, or from a donor – known as allogeneic cell therapies – can be used.
Although cell therapies in the form of bone marrow transplants have been used to treat rare forms of blood cancer since the 1950s, most commercially available cell-based therapies today have been approved within the past decade.
To date, 27 cell therapies have been approved by the United States Food and Drug Administration (FDA) for the treatment of multiple indications, including various forms of cancer, inherited metabolic disorders and immune system disorders.1 Many more are likely to be submitted for approval soon, given the over 1,600 ongoing clinical trials for cell therapies registered with ClinicalTrials.gov.2
In this article, we take a look at how researchers are tackling some of the challenges that are preventing cell therapies from being more widely used, paying particular attention to cellular immunotherapies for cancer and induced pluripotent stem cells (iPSC) for regenerative medicine. We also outline some advances in manufacturing and regulatory approval processes that will help expand patient access to innovative, life-changing treatments.
Non-stem cell-based therapies
These types of therapies involve somatic cells from a patient or a donor, such as fibroblasts, pancreatic islet cells or immune cells. Examples include allogeneic cultured keratinocytes for the treatment of adults with thermal burns and allogeneic pancreatic islet cells for people with type 1 diabetes who are unable to maintain healthy blood sugar levels despite thorough diabetes management and education.
The most commonly used somatic cells are T cells, white blood cells that are critical for triggering an immune response to pathogens, allergens and tumors. The first chimeric antigen receptor (CAR) T cell to be approved by the FDA in 2017 was invented by Professor Carl June at the University of Pennsylvania. This CAR T-cell therapy – known as tisagenlecleucel (Kymriah®) – can be used for the treatment of certain pediatric and young adult patients with advanced chronic lymphocytic leukemia.
Currently, there are six FDA-approved CAR T-cell therapies for the treatment of various lymphomas and myeloma. These cellular immunotherapies involve collecting T cells from the patient’s blood and modifying them genetically in the laboratory so that they make CARs that track down cancer-associated antigens. When re-infused into the patient, the modified T cells attach to and kill the cancer cells, thereby helping to clear the cancer from the body.
Dr. Neil Sheppard, a director in Prof. Carl June’s laboratory, highlights the advantages of cellular immunotherapies for cancer: “We know it is possible for the immune system to completely cure us of even advanced diseases, including cancer, under the right circumstances and potentially without damaging healthy tissues,” he explains.
While he acknowledges that a lot of work remains to be done to realize the full potential of these therapies, he is optimistic about progress. “With various genetic, epigenetic and RNA-editing tools the promise of cellular immunotherapies is within our grasp,” he says.
Next-generation cellular immunotherapies
Despite the remarkable efficacy of CAR T-cell therapies against B-cell malignancies, patients can experience recurrence if the cancer cells stop expressing the targeted antigen, and CAR T-cell therapies have also shown limited efficacy against solid tumors.3,4
To address these challenges, Sheppard and others are pursuing several approaches. These include developing CAR T cells that target multiple antigens to prevent immune escape and using alternative cell types such as natural killer (NK) cells, which do not mediate graft-versus-host disease and might be easier to use in an allogeneic setting.5
“Solid tumors remain a challenge,” says Sheppard. “To achieve CAR T efficacy in solid tumors, target antigens such as claudin 6 and claudin 18.2 are being utilized, sometimes in conjunction with vaccination with the antigen to boost CAR T-cell proliferation and activity.”
There is growing evidence that intratumoral injection of CAR T cells can have a greater effect compared to intravenous infusion. Moreover, combining CAR T cells with oncolytic viruses that attract CAR T cells to the tumor aids tumor infiltration and strengthens resistance to the immunosuppressive tumor microenvironment.6
The recent approval of the first tumor-infiltrating lymphocyte (TIL) therapy raises new hope for cell therapies in solid cancers.7 TILs have an innate ability to seek out cancer neoantigens, fragments of mutated intracellular proteins that are presented on the surface of cancer cells. One such treatment for unresectable or metastatic melanoma – lifileucel (Amtagvi™) – involves the removal of TILs from patients’ tumor tissue, growing them in bioreactors and re-infusing them into patients to destroy cancer cells expressing these patient-specific antigens.
Stem cell-based therapies
Stem cell treatments play a key role in regenerative medicine as their ability to self-renew and differentiate into specialized cells can be used to replace damaged or diseased cells and restore normal function.
Dr. Shinya Yamanaka’s groundbreaking discovery that adult cells can be reprogrammed into pluripotent stem cells through the expression of embryonic transcription factors offers researchers the opportunity to generate patient-specific regenerative cell therapies without having to rely on controversial embryonic stem cells or worry about immune rejection.8
Since then, great progress has been made in generating and differentiating iPSCs. “iPSCs are remarkably useful, and in a class by themselves,” says Professor Jeanne Loring, founding director of the Center for Regenerative Medicine and emeritus professor at the Scripps Research Institute in La Jolla, California. “They are the only non-cancer cells that can continue to divide indefinitely in a culture dish, and retain the ability to give rise to every cell type in the body.”
Loring’s research focuses on developing iPSC-based therapies for Parkinson’s disease, multiple sclerosis and autism. She believes these therapies hold great promise for conditions known to be caused by the loss of specific cell types. However, there are still several obstacles associated with iPSC-based therapies that need to be addressed before they can enter clinical trials.
“The development of iPSC-derived cell therapies requires cutting-edge technologies in multiple areas – from making the stem cells and differentiating them into the right cell type in culture, to determining the best stage of differentiation for transplanting the cells and sophisticated genomics and bioinformatics, with machine learning to make sure the cells are at the right stage and that they don’t contain dangerous mutations,” Loring explains.
In her opinion, technology is key to overcoming these challenges. “This is a field where all of the work has been hands-on with highly skilled people, which makes it very expensive,“ she says. “The use of AI to make decisions about the culture of the cells and new genomics methods that ensure the integrity of the genomes of cells before they are transplanted, are just a couple of examples of ways to advance cell therapies to the clinic.”
Manufacturing innovations
In addition to sharing the main challenges of drug development, such as the need to demonstrate safety, target engagement and pharmacological activity, cell therapies face unique challenges. “The manufacturing process for CAR T-cell therapy greatly affects the potency of cell therapy product,” says Sheppard. His team is exploring how to scale up their cell manufacturing process in the laboratory into a large-scale good manufacturing process (GMP) needed for clinical trials or even a commercial scale process designed to serve thousands of patients each year.
So far, they have learned that, with patient-specific immune cells, the longer the duration of the cell manufacturing process and the more manipulation steps involved, the lower the potency of the emerging cell. “The evidence shows that CAR T-cell products made in manufacturing processes as short as one to three days have superior activity once inside the human body compared to those made over nine days or more.”
In the not-too-distant future, Sheppard envisages the creation of self-contained GMP machines where a patient’s T cells are loaded and the chosen type of CAR T-cell therapy is produced at the press of a button in a hospital setting. “I imagine the future of CAR T-cell therapy production being as simple from the prescriber’s perspective as using the office coffee machine – load the desired sachet (gene vector), water (reagents) and a cup (cells), press the button and your work is done.”
Speeding up production of stem cell-based therapeutics
Since 2003, the UK’s Stem Cell Bank (UKSCB) has been supporting the development of pluripotent stem cell-derived therapies and driving improvements in the manufacturing process to produce quality-controlled pluripotent stem cell lines (iPSCs and human embryonic stem cells, or hESCs) that can be differentiated into specialized cell types for tissue regeneration. At the 20th anniversary of the cell repository last October, Dr. Lee Carpenter, the head of the UKSCB, highlighted automation and machine learning as being key to accelerating the safe manufacture of stem cell-derived therapies and giving patients faster access to potentially life-saving treatments.9
The UKSCB has demonstrated, using a robotic system, that clinical-grade pluripotent stem cell production is possible and is currently comparing the automated process to manual production. The use of automation, robotics and modular production platforms will not just help reduce reliance on manual operations and variability in process performance, it will also enable scalability and drive down costs. “There is a race to develop the instrumentation to automate stem cell-derived cell therapy manufacturing,” says Loring.
Streamlining the approval process
The final hurdle when developing cell therapies is obtaining regulatory approval. The review of a New Drug Application (NDA) by the FDA can take up to a year, significantly delaying the commercialization of a new therapy.10
Cell therapies are evolving quickly, and so too must the regulations. Regulatory agencies are adapting to the unique challenges of cell therapies by implementing pathways aimed at expediting assessments, such as the FDA's Regenerative Medicine Advanced Therapy (RMAT) designation and the European Medicines Agency (EMA)'s Priority Medicines (PRIME) scheme.11 In addition, the FDA is on a recruitment drive to hire more staff in its Office of Therapeutic Products to tackle the increasing workload.12
Efforts to increase the dialog between regulators, industry and academia to harmonize regulatory standards are also starting to bear fruit. The benefits of international programs to build regulatory capacity and share information are evidenced by multinational approvals of CAR T-cell therapies.13 As the cell therapy pipeline continues to expand, increasing the alignment of regulatory pathways across countries will be crucial to facilitating access to new transformative treatments to patients around the world.
About the interviewees:
Dr. Neil Sheppard is the director of the Therapeutic Innovation in Natural Killer cells (THINK) lab, which focuses on improving the therapeutic application of NK cells in cancer. As part of Prof. Carl June's team, he is also overseeing the development of combination strategies for clinical-stage CAR T-cell therapies. Sheppard joined Penn Medicine’s Center for Cellular Immunotherapies in 2019 and has over 20 years experience of working on immunotherapies in academia, pharma and biotechnology companies.
Dr. Jeanne Loring is professor emeritus at The Scripps Research Institute, where she was the founding director of the Center for Regenerative Medicine. She is an internationally recognized authority in stem cell research and has received multiple awards for her accomplishments as a scientist, educator and advocate for evidence-based patient therapies. In 2018 she co-founded the biotechnology company Aspen Neuroscience to develop personalized cell therapies for Parkinson’s disease.
References:
1. Approved Cellular and Gene Therapy Products. FDA. Published online March 18, 2024. Accessed April 26, 2024. https://www.fda.gov/vaccines-blood-biologics/cellular-gene-therapy-products/approved-cellular-and-gene-therapy-products
2. Active clinical trials of cellular therapies. clinicaltrials.gov. https://clinicaltrials.gov/search?intr=cell%20therapy&aggFilters=status:act
3. Cappell KM, Kochenderfer JN. Long-term outcomes following CAR T cell therapy: what we know so far. Nat Rev Clin Oncol. 2023;20(6):359-371. doi: 10.1038/s41571-023-00754-1
4. Hou AJ, Chen LC, Chen YY. Navigating CAR-T cells through the solid-tumour microenvironment. Nat Rev Drug Discov. 2021;20(7):531-550. doi: 10.1038/s41573-021-00189-2
5. Myers JA, Miller JS. Exploring the NK cell platform for cancer immunotherapy. Nat Rev Clin Oncol. 2021;18(2):85-100. doi: 10.1038/s41571-020-0426-7
6. Daei Sorkhabi A, Mohamed Khosroshahi L, Sarkesh A, et al. The current landscape of CAR T-cell therapy for solid tumors: Mechanisms, research progress, challenges, and counterstrategies. Front Immunol. 2023;14. doi: 10.3389/fimmu.2023.1113882
7. Mullard A. FDA approves first tumour-infiltrating lymphocyte (TIL) therapy, bolstering hopes for cell therapies in solid cancers. Nat Rev Drug Discov. 2024;23(4):238-238. doi: 10.1038/d41573-024-00035-1
8. Takahashi K, Yamanaka S. Induction of pluripotent stem cells from mouse embryonic and adult fibroblast cultures by defined factors. Cell. 2006;126(4):663-676. doi: 10.1016/j.cell.2006.07.024
9. Patients could have faster access to ground-breaking stem cell treatment with manufacturing innovation. GOV.UK. Published online October 11, 2023. Accessed April 29, 2024. https://www.gov.uk/government/news/patients-could-have-faster-access-to-ground-breaking-stem-cell-treatment-with-manufacturing-innovation
10. Rotolo A, Chabannon C, Gramignoli R. Identification of hurdles in the development of cell-based therapies. Cytotherapy. 2020;22(2):53-56. doi: 10.1016/j.jcyt.2019.12.009
11. Drago D, Foss-Campbell B, Wonnacott K, Barrett D, Ndu A. Global regulatory progress in delivering on the promise of gene therapies for unmet medical needs. Mol Ther Methods Clin Dev. 2021;21:524-529. doi: 10.1016/j.omtm.2021.04.001
12. Establishment of the Office of Therapeutic Products. FDA. Published online March 16, 2023. Accessed April 29, 2024. https://www.fda.gov/vaccines-blood-biologics/cellular-gene-therapy-products/establishment-office-therapeutic-products
13. Arcidiacono J. International Harmonization for Cell and Gene Therapy Products. In: Galli MC, ed. Regulatory Aspects of Gene Therapy and Cell Therapy Products: A Global Perspective. Springer International Publishing; 2023:235-240. doi: 10.1007/978-3-031-34567-8_14