Water Analysis: A Pollution Solution?
We’re all familiar with the importance of water, but how do we analyze it? And how can this knowledge be used?
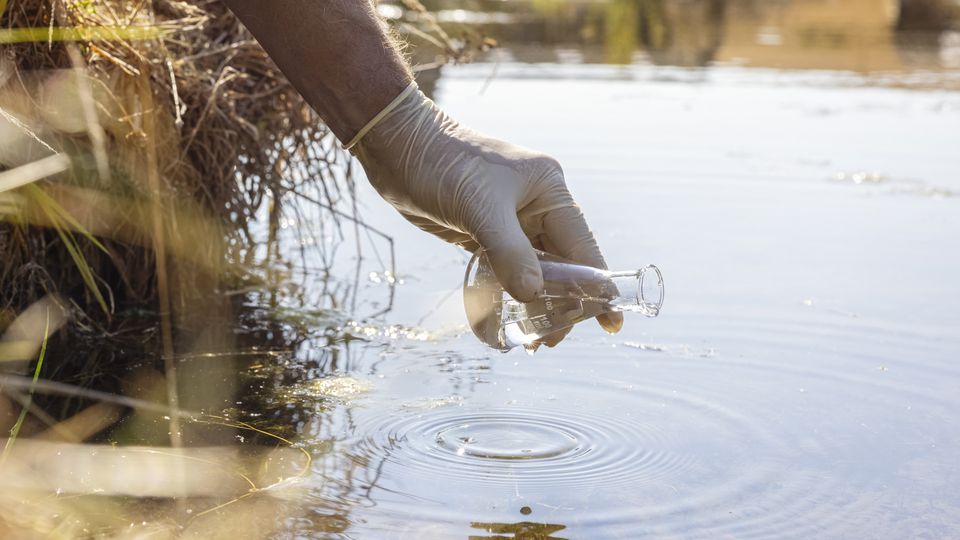
Complete the form below to unlock access to ALL audio articles.
Water is an essential ingredient for life on Earth – and possibly beyond. For modern humans, water represents a means to hydrate, wash, cook, clean and even to dispose of our waste. But our activities are not without consequences. Every headline about pollutants like microplastics and forever chemicals (namely per- and polyfluoroalkyl substances) is a daunting reminder that our presence on this planet has a direct impact on the quality of its water.
Understanding this impact could help us to improve water quality and avoid the potential adverse effects of contaminants. But how big is the problem? What tools are used to quantify it? And how can this knowledge help us?
The tools of the trade
“There are over 200 parameters that we can analyze when assessing water quality and most of these are source- and location-specific,” said Dr. Sarper Sarp, a senior lecturer at Loughborough University.
Among these parameters are a wide variety of contaminants. Though many researchers have focused on traditional pollutants (like nutrients and heavy metals), so-called emerging pollutants are now taking center stage.
“There isn’t enough information about emerging pollutants and their impact,” explained Prof. Rachel Gomes, a professor of water and resource processing at the University of Nottingham. “These include pharmaceuticals, microplastics, and microorganisms that have developed resistance to commonly used antimicrobials.” The impact of these pollutants is widely documented: microplastics were identified in human breastmilk in 20221 and antimicrobial resistance caused an estimated 1.27 million deaths globally in 2019.2
The choice of technique used for water analysis is largely driven by the application and target. A combination of gas (GC) or liquid chromatography (LC) with mass spectrometry (MS) can prove effective for targeted and untargeted contaminant identification, but each approach has its own strengths (and preferred targets). MS variants, like matrix-assisted laser desorption/ionization time-of-flight MS, can also be applied to study microorganisms in water sources.3
Some contaminants might be identified via Raman spectroscopy, which studies the vibrational modes of molecules and requires minimal sample preparation. Biosensors, which convert biological signals into a measurable response, also represent a viable option with high sensitivity and selectivity and the potential for miniaturization.4
Of course, other techniques – like genomic methods – are available for specific applications. And, as may be expected, our analytical capabilities have changed (and will continue to change) over time. As Sarp said: “We used to be able to detect contaminants at the parts per million level in water; now we can study them at parts per trillion. The driving force is knowledge – and the fact that some of these chemicals can be toxic at extremely low concentrations.”
Life in plastic
Of all the analytes that scientists study in water, plastics have probably received the greatest level of media attention in recent years. Worldwide familiarity with microplastics is testament to this coverage, but how problematic are they?
“Microplastics were a big focus in research a few years ago,” Sarp explained. “But microplastics often leave the body without a negative impact because of their chemical inertness and large size. I wondered if we could study nanoplastics instead, which are small enough to penetrate cell walls and cause toxicity. We did so by combining nanofiltration with pyrolysis GC-time-of-flight MS (considered the best technique to identify specific plastics). Our approach made the analysis of nanoplastics in water possible for the first time.”5
But simply detecting these plastics is not enough. “The wider context around plastics is also important to consider,” Gomes said. “What type of polymer are we looking at? Are they weathered or pristine (unweathered, or as manufactured)? What is their size and distribution? Not all microplastics are created equal. How do their individual qualities influence their fate and impact? And are the samples analyzed truly representative of the wider water environment being studied? These factors must be explored to gain a full understanding of the problem.”
Combatting COVID
Wastewater represents a powerful analytical target because an estimated 2.1 billion people are connected to wastewater treatment processes around the world.6 Many scientists have used – and are still using – this water as an opportunity to study COVID-19.
Rolf Halden and colleagues began using the quantitative polymerase chain reaction and metagenomic approaches to study SARS-CoV-2 particles in wastewater on a regular basis in Tempe, Arizona, during the early days of the pandemic. The hope was to identify local infection hotspots, and the online dashboard still boasts results from these analyses.7
A study led by clinician Dr. Tanya Monaghan at the University of Nottingham accomplished a similar feat in Nagpur, India. The team, which included Gomes, applied RNA sequencing to retrospectively measure SARS-CoV-2 and other zoonotic viruses from a total of 140 sample sites and identified SARS-CoV-2 in 59% of the locations studied (though the levels of virus varied massively between sites).8 They also detected several infectious agents in wastewater for the first time – including Jingmen tick, chikungunya, and rabies viruses – but Hepatitis C was the most common virus detected. Studies like this provide an opportunity to assess the health status of large populations and can help to direct interventions against the diseases present.
Sarp’s contributions to the fight against COVID-19 via wastewater analysis again focused on nanoplastics. “When the pandemic started, we shifted our focus to facemasks and whether they emit nanoplastics once disposed of,” he said. “To our surprise, we found that each mask released thousands of nanoparticles, some of which were laced with heavy metals like arsenic, lead and copper. The potential impact of these findings could be huge.”
What’s next?
Water analysis is essential to protect people and our planet, but we have a long way to go to truly understand and ensure the quality of our water. Part of the problem is the limitations of our current knowledge and technologies.
“We need to answer a number of key questions,” Gomes explained. “Which emerging pollutants should be treated as a priority? Can current treatment technologies remove them? How can we improve these technologies where needed, and what new technologies or strategies might be necessary?” Essential technological advances will include the development of cheap and mobile analytical equipment, as well as remote sensing capabilities and end user-based monitoring.
Another important issue to address will be inequity in water analysis between geographies. “Analytical approaches and their availability need to be more inclusive,” Gomes said. “Access to LC-MS/MS instrumentation is limited in some countries and existing machines tend to become outdated very quickly. This is driven partly by the need for ever-decreasing detection limits. Determinations of environmental risk using predicted no effect concentrations (PNECs; the level at which a contaminant is thought to have no negative impact) could also be modified to be more geographically equitable. Current PNECS tend to focus on European aquatic species, but are also applied to other regions where they may not be relevant. Ways to reduce contamination levels in water without the need for expensive technologies and processes are needed, too.”
Ambitious targets like the 9% reduction in microplastics by 2040 proposed by the European Commission9 paint a picture of a bright (and clean) future. Perhaps suggestions like those above can help us to achieve it.
About the interviewees
Dr. Sarper Sarp is a senior lecturer in the School of Architecture, Building and Civil Engineering at Loughborough University. His research focuses on water quality, micropollutant monitoring in water, and water treatment systems.
Prof. Rachel Gomes is a professor of water and resource processing in the Faculty of Engineering at the University of Nottingham. Her research focuses on wastewater and reuse, with an emphasis on pollutants, water quality, and waste valorization opportunities.
Dr. Tanya Monaghan is a clinical associate professor and honorary consultant in gastroenterology in the Faculty of Medicine and Health Sciences at the University of Nottingham. Her current research interests include developing wastewater-based epidemiological approaches to understand population health in East Africa and India.
References
1. Ragusa A, Notarstefano V, Svelato A, et al. Raman microscopy detection and characterisation of microplastics in human breastmilk. Polymers. 2022. doi: 10.3390/polym14132700
2. Antimicrobial resistance. World Health Organization. https://www.who.int/news-room/fact-sheets/detail/antimicrobial-resistance. Published November 21, 2023. Accessed March 19, 2024.
3. Jiang W, Lin L, Xu X, et al. A critical review of analytical methods for comprehensive characterization of produced water. Water. 2021. doi: 10.3390/w13020183
4. Zakir Hossain SM, Mansour N. Biosensors for on-line water quality monitoring – a review. Arab J Basic Appl Sci. 2019. doi: 10.1080/25765299.2019.1691434
5. Sullivan GL, Delgado Gallardo J, Jones EW, Holliman PJ, Watson TM, Sarp S. Detection of sub-micron (nano) plastics in water samples using pyrolysis-gas chromatography time of flight mass spectrometry (PY-GCToF). Chemosphere. 2020. doi: 10.1016/j.chemosphere.2020.126179
6. Hart OE, Halden RU. Computational analysis of SARS-CoV-2/COVID-19 surveillance by wastewater-based epidemiology locally and globally: Feasibility, economy, opportunities and challenges. Sci Total Environ. 2020. doi: 10.1016/j.scitotenv.2020.138875
7. Biomarker: COVID-19. Tempe Wastewater BioIntel Program. https://wastewater.tempe.gov/pages/biomarker-covid19. Accessed March 19, 2024.
8. Stockdale SR, Blanchard AM, Nayak A, et al. RNA-Seq of untreated wastewater to assess COVID-19 and emerging and endemic viruses for public health surveillance. Lancet Reg Health Southeast Asia. 2023. doi: 10.1016/j.lansea.2023.100205
9. Urban wastewater. European Commission. https://environment.ec.europa.eu/topics/water/urban-wastewater_en. Accessed March 19, 2024.