Viral Clearance in a Downstream AAV Process
App Note / Case Study
Published: February 28, 2024
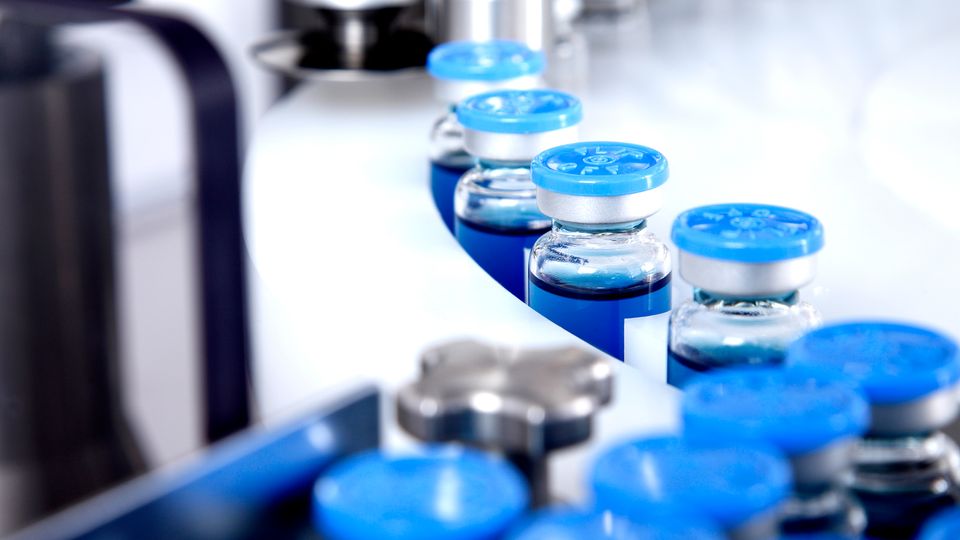
Credit: iStock
Over the last 10 years, adeno-associated virus (AAV) vectors have emerged as primary gene delivery tools, with hundreds in clinical trials and preclinical development.
As these candidates progress, substantial focus is placed on refining process development and manufacturing for viral vectors, performing inactivation and filtration steps to minimize impurities without compromising product yield.
This application note shows how chromatographic modes of separation can achieve the necessary levels of virus removal while preserving the integrity of the AAV product.
Download this app note to discover:
- How to achieve robust viral clearance across various viruses
- A method that demonstrates high overall process log reduction values
- An accurate and economic prediction model for AAV purification processes
*Originally published and cited from Bioprocess International
Viral Clearance in a Downstream AAV Process Case Study Using a Model Virus Panel and a Noninfectious Surrogate Michael Winkler, Mikhail Goldfarb, Shaojie Weng, Jeff Smith, Susan Wexelblat, John Li, Alejandro Becerra, Sandra Bezemer, Kevin Sleijpen, Aleš Štrancar, Sara Primec, Romina Zabar, April Schubert, Akunna Iheanacho, and David Cetlin Product Focus: Viruses Process Focus: Downstream processing Audience: Process engineers, analytical, manufacturing Keywords: Monoliths, affinity chromatography, adenoassociated virus, TCID50, Immuno-qPCR, SPR analysis Level: Intermediate Over the past decade, adenoassociated virus (AAV) vectors have become established as leading genedelivery vehicles. In 2017, the pipeline for gene therapies included 351 drugs in clinical trials and 316 in preclinical development (1–4). As those candidates advance, significant efforts are being made in process development and manufacturing for viral vectors, with the overall goal of reducing process impurities while maintaining the highest possible process yield. To address that goal, industry suppliers have developed innovative AAV-specific separation technologies. Thermo Fisher Scientific’s POROS CaptureSelect AAVX affinity resin provides a capture method for a number of natural and synthetic AAV serotypes irrespective of the expression system used to produce them. By leveraging a proprietary recombinant camelid antibody technology immobilized onto the highly permeable POROS backbone, the resin achieves a fine-tuned specificity for AAV recognition with an increased surface area and capacity for AAV binding. The significant impurity reduction benefits and rapid scalability of this affinity resin have led to its incorporation into several noted AAV downstream process designs. BIA Separations (now part of Sartorius AG) has developed and commercialized CIMmultus QA monoliths, which have been cited in several AAV downstream processes for their ability to separate empty and full virus particles effectively. Monolithic supports represent a unique type of stationary phase for liquid chromatography, bioconversion, and solid-phase synthesis. Aside from increased processing speed, monolithic flow-through pores (channels) also provide easy access for large molecules, which supports both purification and depletion of nanoparticles such as plasmid DNA (pDNA) molecules and AAV particles. One elusive aspect of AAV process development is viral clearance (VC). As outlined in the ICH Q5A guidelines, VC validation is a key regulatory requirement governing all recombinant biopharmaceuticals (5). According to these guidelines, the risks of viral contamination should be assuaged by a three-pronged approach: prevent, test, and remove. Over the past few decades, Similarity in structures of adenoassociated virus serotype 8 (top) and minute virus of mice (bottom). PROTEIN DATA BANK (HTTPS://WWW.RCSB.ORG)April 2021 19(4) BioProcess International 39 certain VC strategies for monoclonal antibodies (MAbs), such as low-pH inactivation and nanofiltration, have become standard for most downstream processes. However, because AAVs are viruses themselves (within the family Parvoviridae), it may not be possible to apply the same purification strategies to them that have served so well in MAb processes. As a result, the gene-therapy industry may depend increasingly on chromatographic modes of separation to demonstrate sufficient viral clearance. In the study reported herein, we addressed viral removal by performing scale-down–model spiking studies and measuring VC using a clinically relevant AAV8 downstream purification process. The two-step chromatography process begins with affinity capture using POROS CaptureSelect AAVX affinity resin followed by anion-exchange polishing using a CIMmultus QA monolith. We selected as spiking agents a wide range of viruses with different sizes, molecular makeups, and physiochemical properties. For DNA viruses, we used enveloped pseudorabies virus (PRV) and nonenveloped minute virus of mice (MVM). For RNA viruses, we used enveloped xenotropic murine leukemia virus-related virus (XMuLV) and nonenveloped reovirus type 3 (Reo-3). We also included human contagions hepatitis A (HAV) and herpes simplex virus 1 (HSV-1) based on a risk assessment of possible adventitious virus contaminations with a HEK293 human-derived producer cell line used for upstream production. For the benefit of future process developers who may wish to perform similar VC testing on their own purification process steps but lack the ability to conduct such resource-intensive studies, we assessed the MockV MVM kit from Cygnus Technologies, LLC, as an economical and rapid means to generate predictive MVM clearance data (6). We used the kit in parallel with MVM spiking experiments throughout our study. Materials All AAVs used for these viral clearance studies were produced at representative scale by a platform process at REGENXBIO Inc. Production of Virus and Mock Virus Particles (MVPs): Viruses were propagated and purified by Texcell NA of Frederick, MD, according to standard protocols. Noninfectious MVM-MVPs were assembled after expression of the major MVM capsid protein (VP2) in a baculovirus expression-vector system (BEVS) using Spodoptera frugiperda 9 (Sf9) cells at Cygnus Technologies. Particles were purified with affinity and ion-exchange chromatography (IEC). Transmission electron microscopy (TEM) was used to verify MVP morphology, size, and concentration. Chromatography Products: Thermo Fisher Scientific supplied prepacked, 5-mL POROS CaptureSelect AAVX affinity resin columns; BIA Separations provided scale-down CIMmultus QA monolith devices in 4-mL and 8-mL diameters. Control POROS resins were custom made by Thermo Fisher Scientific with identical base beads to Table 1: Adenoassociated virus (AAV) process spiking runs for xenotropic murine leukemia virus (XMuLV), hepatitis A (HAV), reovirus type 3 (Reo-3), pseudorabies virus (PRV), herpes simplex virus 1 (HSV-1), minute virus of mice (MVM), and the MVM mock virus particle (MVM-MVP) Spiking Agent POROS CaptureSelect AAVX CIMmultus QA Center Point Worst Case Center Point Worst Case Run 1 Run 2 POROS Alternative Ligand POROS Base Matrix AAV Null Load XMuLV ✓ ✓ ✓ ✓ ✓ ✓ ✓ HAV ✓ ✓ ✓ ✓ Reo-3 ✓ ✓ ✓ ✓ PSV ✓ ✓ ✓ ✓ HSV-1 ✓ ✓ ✓ ✓ MVM ✓ ✓ ✓ ✓ ✓ ✓ ✓ MVM-MVP ✓ ✓ ✓ ✓ ✓ ✓ ✓ ✓ Figure 1: (a) Immuno-quantitative real-time polymerase chain reaction (Immuno-qPCR) assay; (b) Immuno–qPCR standard curve Mock virus particle (MVP) Anti-MVP antibody conjugated to target DNA Anti-MVP capture antibody Cycle Threshold (Ct) Value MVM-MVP/mL 40 30 20 10 0 104 106 108 1010 y = –0.915ln(x) + 43.542 R² = 0.9815 A B Figure 2: Viral clearance using affinity resin; see Table 1 for full virus names Log Reduction Value XMuLV MVM Reo-3 HAV PRV HSV-1 7 6 5 4 3 2 1 0 Manufacturing Center-Point Process Worst-Case Process 6.4 4.6 4.4 3.6 2.7 2.5 4.9 5.0 4.0 3.8 3.1 3.640 BioProcess International 19(4) April 2021 the affinity resin used but incorporating either an alternative VHH ligand specificity (nonbinding for AAVs) or no VHH functionalized ligand. Methods Study Design: To assess the robustness of the affinity resin and monolith polishing step within REGENXBIO’s downstream process (7), we selected “center-point” and “worst-case” processing parameters for our viral clearance spiking experiments (Table 1). For each run, we spiked in-process AAV material with model viruses or MVM-MVPs to a target of 10.0 log10 MVM-MVP/mL and processed accordingly. For affinity resin center-point and alternative-ligand runs, we loaded 150 mL of spiked material according to standard manufacturing load ratios and residence time. For worst-case conditions, we loaded 200 mL of spiked material (133% of the target) and decreased the flow rate to lengthen residence time to 170% of the centerpoint target. For the monolith polishing study, we applied center-point load volumes of 90 mL to 8-mL monoliths and 65 mL to 4-mL monoliths for worst case — except for the MVP- and XMuLVspiked runs. For those, we loaded 45 mL and 65 mL onto 4-mL columns, respectively, for the center-point and worst-case conditions. Samples were collected from each run during each step phase (flowthrough, wash, and so on). We analyzed the virus samples immediately with a 50% tissue culture infectious dose (TCID50) assay or quantitative real-time polymerase chain reaction (qPCR). MVM-MVP samples were stored at –80 °C before Immuno-qPCR analysis (described below). From those results, we determined log reduction values (LRVs) using a standard calculation (5). During this study, we performed affinity-capture experiments to probe potential nonspecific binding interactions (Table 1). Both AAV-null (produced by pooling the flow-through fractions of previous AAVX runs) and AAV8-containing load materials were spiked with model virus, then affinity purified using center-point conditions and compared for viral clearance. Additionally, we evaluated interactions between viruses and base beads by performing AAV8 center-point runs using the POROS base matrix without a functionalized VHH ligand; we evaluated virus–VHH ligand interactions using a POROS resin with an alternative VHH ligand specificity to the Fc portion of MAbs that cannot bind AAVs. We applied an orthogonal test method — surface plasmon resonance (SPR) — to confirm the specificity of the AAVX ligand for AAV. For that, a biotinylated AAVX VHH ligand was immobilized onto a detection surface so that binding sensograms could be generated by injection of free MVM-MVP or AAV. Analytical Assays and LRV Determinations: Texcell scientists quantified infectious titer of XMuLV using a validated plaque-forming infectivity assay. They quantified HAV, Reo-3, HSV-1, and MVM using validated TCID50 infectivity assays. PRV was quantified with a validated qPCR assay. From those titer determinations, we calculated LRVs by a standard method (5). To analyze the concentration of noninfectious MVM-MVP within each sample, Cygnus Technologies scientists performed an Immuno-qPCR assay (Figure 1a) as described elsewhere (8). In brief, samples were added to microwells coated with an anti–MVMMVP capture MAb. After incubation and washing, a DNA-conjugated anti–MVMMVP detector MAb was added. Following another incubation and washing step, a dissociation buffer was added to each Figure 4: Characterization of nonspecific interactions based on log reduction values (LRVs) for xenomorphic murine leukemia virus (XMuLV) and minute virus of mice (MVM) XMuLV MVM LRV 7 6 5 4 3 2 1 0 Control Alternative VHH Ligand AAV-null POROS Base Matrix Figure 3: Log reduction value (LRV) determinations for affinity resin runs spiked with minute virus of mice (MVM) and noninfectious MVM mock virus particles (MVM-MVPs) LRV 5 4 3 2 1 0 4.35 5.035 3.58 4.07 3.79 4.28 MVM MVM-MVP Center Point Alternate Ligand Worst Case Table 2: Affinity resin data from experiments with minute virus of mice (MVM) and noninfectious MVM mock virus particles (MVM-MVPs) Run Type Phase Total Particles (log10) Percentage of Particles MVM MVM-MVP MVM MVM-MVP Run 1 Run 2 Run 1 Run 2 Center Point Load 8.1 12.3 12.2 NA NA NA FT 7.9 12.0 12.0 66.1% 52.6% 67.0% Wash 1 6.1 10.0 9.7 1.0% 0.5% 0.3% Wash 2 5.4 11.3 11.2 0.2% 10.4% 10.4% Wash 3 4.7 8.7 8.7 0.0% 0.0% 0.0% Elution 3.8 7.4 7.0 0.0% 0.0% 0.0% CIP 5.0 6.9 6.7 0.1% 0.0% 0.0% Worst Case Load 7.9 11.9 NT NA NA NA FT 7.6 11.8 55.0% 79.1% Wash 1 NT 9.9 NA 1.1% Wash 2 NT 11.0 NA 14.3% Wash 3 NT 9.0 NA 0.1% Elution 4.3 7.8 0.0% 0.0% CIP NT 6.8 NA 0.0% NT = not tested; NA = not applicable; FT = flow-through fraction; CIP = clean-in-place solutionApril 2021 19(4) BioProcess International 41 well for five minutes. Then 5 µL of sample was transferred from each well to a qPCR plate containing TaqMan primers/probes (Thermo Fisher Scientific) directed against the conjugated DNA. To determine the quantity of particles in unknown samples, threshold cycle (Ct) values were interpolated into a standard curve generated by including a 10-fold dilution series of a known MVM-MVP standard (Figure 1b). From those concentration values, we could calculate MVM-MVP LRVs for each experiment. Results and Discussion Viral clearance strategies for AAV downstream processes are limited by difficulty in performing viral inactivation and filtration steps without also inactivating or removing the AAV product. Therefore, chromatographic modes of separation are paramount to achieving the desired levels of removal for viruses of concern. To complicate matters further, MVM (a small, nonenveloped virus used internationally as a model spiking agent) is a member of the same parvovirus family as AAV. Morphology and physicochemical properties (size,
Download this App Note for FREE Below
Information you provide will be shared with the sponsors for this content.
Technology Networks or its sponsors may contact you to offer you content or products based on your interest in this topic. You may opt-out at any time.