Smarter Fusion Detection for Cancer Diagnostics
App Note / Case Study
Published: June 28, 2024
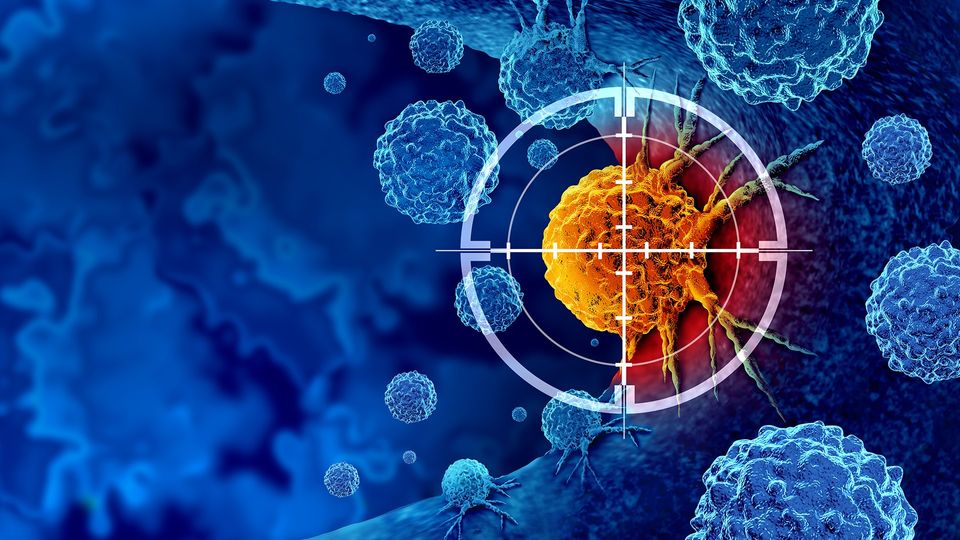
Credit: iStock
Fusion transcripts, resulting from gene fusions, play a significant role in tumorigenesis and the resulting proteins are prime targets for cancer diagnostics and therapies.
Not all fusion genes are readily detected at the DNA level from relevant fusion genes which modify the RNA and protein function, especially in limited tumors specimens. Thus, RNA analysis offers better detection sensitivity, which is critical when working with FFPE tumor samples with degraded material quality.
This app note highlights a robust targeted RNA enrichment workflow that integrates automation to expedite library preparation and data analysis.
Download this app note to discover a method that:
- Enhances the sensitivity of fusion detection
- Enables precise tumor classification
- Accurately informs treatment decisions with actionable insights
A Smarter Way to Fish For Fusions Genetic
Diagnostics
Application Note
Tumor Development
Cancer is the second most frequent cause of death worldwide. Tumor development begins when the DNA of a single cell changes, causing the
cell to multiply abnormally. DNA changes, called mutations, happen frequently and are typically corrected by the cell’s DNA repair systems. As an
additional safety, failed DNA repair leads to the death of the mutated cell. In very rare cases, DNA repair fails, and the cell survives. If the mutation
leads to altered function of a protein involved in growth control, the cell starts to multiply uncontrollably, and a tumor emerges.
The Role of Fusion Transcripts
Besides single nucleotide and copy number variants, many types of
cancer are caused by gene fusions. Fusion events bring together
parts of two different genes, often changing the activity of one of the
partners. For example, a protein that is only active in the presence of
an activating ligand in healthy cells may be permanently switched
on after either losing the regulatory domain controlled by this ligand
or by fusion to a gene that supports constitutive activation. Such
“always-on” proteins then activate downstream pathways that regulate
cell growth, survival, proliferation, and apoptosis, which in turn leads to
carcinogenesis1
. Examples are the tyrosine kinases ALK, ROS1, RET,
FGFR1/2/3, BRAF, EGFR, and NTRK1.
Kinases are excellent therapy targets since the fusion is usually the
tumor driver and thus present in every cancer cell. Drugs have been
approved for all solid tumors with NTRK1/2/3 fusion, for lung cancers
harboring ALK or ROS1 fusion, in cholangiocellular carcinoma and
bladder cancer harboring FGFR alterations, and in all solid tumors
with RET fusion. Other approved and new drugs are in clinical trials for
tumors harboring gene fusions, e.g., Dabrafenib + Trametinib + HCQ in
brain tumors with BRAF fusion, Belvarafenib for all solid tumors with
BRAF fusion, or Zenocutuzumab for certain tumor entities with NRG1
fusion. Beyond therapy, fusions in solid tumors have also gained importance in diagnosis and tumor classification, especially in sarcomas2,3,4,5.
Fusion Detection from RNA
Fusion transcripts result from structural variants on the DNA level such
as translocations, inversions, or deletions. Their breakpoints are mostly
situated in very large intronic regions, with multiple possible breakpoint locations resulting in the same fusion transcript on the RNA level.
Intronic sequences are especially prone to translocations if the intronic
sequence is non-unique in the genome. This makes both the design of
DNA enrichment panels and the detection of translocations from these
regions challenging. In addition, for clinically relevant splice variants
such as the MET exon 14 skipping variant, the underlying variants on the
DNA level remain mostly undetected.
Conversely, not all fusion genes detected at the DNA level form a relevant
fusion gene at the RNA and protein levels. This is especially the case in
chromosomally highly unstable tumors, where there is an accumulation of
putative fusion genes at the DNA level. Most of them are not transcribed or
break the reading frame, so no meaningful fusion protein is formed.
Analysis on the RNA level thus is both more sensitive and allows for
much better determination of the biological consequence of fusions.
Using targeted enrichment, a focused analysis is possible. By including
probes that span fusion breakpoint sequences, panel enrichment can
significantly increase detection sensitivity with respect to total RNA
sequencing. High sensitivity is crucial when working with clinical FFPE
specimens that combine degraded material quality with potentially low
tumor content.
Gene Fusion Transfer
Chromosomal Translocation
Coding Regions
Break Point
Chromosome 1 Chromosome 2 Derivative Chromosomes Chromosome
Derivative
Chromosome Chromosome
Derivative
Chromosome
Gene Fusion
Gene Fusion
Interstitial Deletion Chromosomal Inversion
Inversion
Coding Regions
Break Point
Gene Fusion Coding Regions
Break Point
somes
Figure 1: Gene fusions result from changes to the chromosomes that join the genomic regions of two genes which were previously at separate locations. Different mechanisms can lead to
such breakpoints. Chromosomal Translocation: Two chromosomes exchange their arms in a translocation, a partial gene from chromosome 1 is joined to a partial gene from chromosome 2;
Interstitial Deletion: The loss of a chromosomal fragment joins two genes previously separated by a large distance; Chromosomal Inversion: The direction of a chromosomal fragment is
changed in an inversion.
Gene Fusion Transfer
Chromosomal Translocation
Coding Regions
Break Point
Chromosome 1 Chromosome 2 Derivative Chromosomes
Gene Fusion Transfer
Chromosomal Translocation
Coding Regions
Break Point
Chromosome 1 Chromosome 2 Derivative Chromosomes
Chromosome
Derivative
Chromosome Chromosome
Derivative
Chromosome
Gene Fusion
Gene Fusion
Interstitial Deletion Chromosomal Inversion
Inversion
Coding Regions
Break Point
Tumor Development
Designing a Therapy-Relevant Fusion
Detection Panel
Our aim in developing the fusion detection panel was to cover the
relevant fusions known to be important for targeted therapeutic drugs,
fusions implicated in resistance to treatment, as well as fusions that are
relevant for tumor diagnosis or subclassification. In addition, we include
important transcript variants such as EGFRvIII and MET exon 14 skipping.
We designed probes spanning known recurrent fusion breakpoints. In
addition, all coding exons of relevant genes are targeted to also detect
fusions where only one partner is known as well as rare breakpoints for
known gene pairs.
We decided to use hybridization enrichment for our panel design for
two main reasons. Firstly, we can capture fusions where we only know
one fusion gene, by designing probes to capture that gene’s exonic
sequence. For fusions with an unknown partner, the molecules thus
captured will extend into that partner’s exonic sequence. Secondly,
hybridization enrichment avoids some issues of amplicon-based strategies, particularly allelic dropout which can happen when primer binding sites have patient-specific mutations and lack of amplification in
highly fragmented samples. To design the hybridization panel, we partnered with Twist Bioscience. The Twist Target Enrichment workflow is
currently one of the best available, giving excellent coverage uniformity
and high capture efficiency.
In addition, we have an automated library preparation pipeline set up
for high-throughput processing of samples, allowing us to process
the fusion panel alongside CeGaT’s ExomeXtra® and other enrichment
panels with no additional process complexity. This means that the
same process is used for patients’ DNA samples (e.g. exomes) and their
RNA samples for fusion detection, and the resulting sequencing-ready
libraries are sequenced alongside one another on the same flowcells.
This streamlined process removes the need to collect a minimum number of RNA samples until a separate library preparation or sequencing
run is feasible. Finally, the technology is agnostic to the downstream
sequencing instrument, which differentiates it from other approaches
that are limited to a specific vendor’s sequencing platform or instrument.
Experimental Workflow
The fusion detection workflow starts with RNA extracted from
fresh-frozen or FFPE tumor material. Following reverse transcription,
10 ng of cDNA from each sample is used as input to the Twist Target
Enrichment workflow using the fusion detection panel. The sequencing-ready libraries are sequenced on the Illumina NovaSeq system
in paired-end mode (PE100), to 5 Gbp (50 million reads) per sample.
The resulting data is analyzed using a pipeline built around the STAR
aligner6
, STAR-Fusion7
, and custom components. The final outputs are
fusion calls and FFPM (fusion fragments per million) values, a normalized abundance score describing the strength of the observed event.
An example implementation of the software pipeline is available as
docker image on our website at www.cegat.com/fusions.
Figure 2: STAR-Fusion visualization of the ETV6-NTRK3 fusion detected in reference material. Top left, transcript variants of ETV6; top right, transcript variants of NTRK3; blue arc, split read
(“junction read”) evidence; purple arcs, split pair (“spanning fragment”) evidence.
Clinical Benefit
Fusion detection is an important part of tumor diagnostics and therapy.
Depending on the tumor entity, fusions may be the main causative
alteration. In prostate cancers analyzed at CeGaT, we find 61% of cases
with TMPRSS2-ERG fusions, and about 28% of glioblastomas show the
EGFRvIII transcript variant. In a retrospective analysis of 1,369 clinical
cases covering 89 different tumor entities, clinically relevant fusions
were detected in 123 (9%) of the cases.
To investigate the benefit of using a targeted enrichment approach, we
compared the results from the RNA panel to fusion transcript detection
from total RNA sequencing in a cohort of 91 patients.
Of those, 27 patients (30%) had clinically relevant fusions detected by
our fusion panel. The enrichment approach has much higher sensitivity
than total RNA sequencing: A third of these events was missed in the
matched total RNA data. For 59 of these patients, we also had exome
sequencing data available, including specific enrichment of intronic
translocation regions. Comparing translocation calls from these with the
fusion calls from the RNA enrichment panel, we find that more than half
of the events (56%) were not detectable from the DNA data.
Conclusion
At CeGaT, we want to offer comprehensive and affordable tumor
diagnostics to our clients. Sensitive detection of fusion transcripts is
highly relevant in tumor diagnostics, with implications for therapy and
prognosis. Targeted enrichment of RNA is a comprehensive and sensitive
method for this task, and the Twist Target Enrichment technology is a
robust workflow that allows us to integrate RNA fusion detection into
automated laboratory processes and sequence samples alongside
exomes without the need for a different library preparation process or
separate sequencing runs. Together with our automated data analysis,
we can accurately analyze patient samples to detect more than
80 therapeutically actionable fusion variants in a high-throughput
manner, allowing us to report clinically actionable results and support
treatment decisions.
Expected Fusion Replicate 1 Replicate 2 Replicate 3
TPM3-NTRK1 88 78 64
LMNA-NTRK1 88 102 80
IRF2BP2-NTRK1 125 132 113
SQSTM1-NTRK1 71 63 51
TFG-NTRK1 67 50 46
AFAP1-NTRK2 93 73 98
NACC2-NTRK2 37 42 27
QKI-NTRK2 33 34 24
TRIM24-NTRK2 38 36 41
PAN3-NTRK2 47 38 35
ETV6-NTRK3 (E5N14)
chr12:12022903-chr15:88576276 165 171 137
ETV6-NTRK3 (E5N15)
chr12:12022903-chr15:88483984 145 160 126
ETV6-NTRK3 (E4N14)
chr12:12006495-chr15:88576276 149 168 137
ETV6-NTRK3 (E4N15)
chr12:12006495- chr15:88483984 159 164 142
BTBD1-NTRK3 88 95 78
Additional calls, max FFPM 2,5 3,8 1,8
Additional calls, FFPM >= 1 4 5 6
Additional calls, FFPM < 1 13 17 18
Table 1: Fusion panel validation results from the SeraCare FFPE NTRK Fusion RNA standard,
sequenced in triplicates. Table shows FFPM (fusion fragments per million) values. All
expected fusions are found with high support. Additional (putative false-positive) calls are
present but with very low support.
Validation and Results
To validate the panel design, we ordered publicly available, certified
reference materials: SeraCare Fusion RNA mix v4, covering 16 fusions
and two transcript variants, and SeraCare FFPE NTRK Fusion RNA,
covering 15 fusions (including 4 alternative events fusing ETV6 and
NTRK3). Both standards were sequenced in triplicates. We also included
five clinical samples, extracting RNA from FFPE material.
All expected fusions were detected in all replicates with high FFPM
values, indicating 100% sensitivity of the fusion panel on this standard,
despite very low RNA quality (RIN=1). A few additional (putative falsepositive) fusion calls were detected, but these were at low levels close
to the detection limit and had a large distance to the scores of the
expected fusions.
Literature
1. Taniue and Akimitsu, Fusion Genes and RNAs in Cancer Development. Noncoding RNA 2021. PMID 33557176.
2. Powers, The ever-changing world of gene fusions in cancer: a secondary gene fusion and progression. Oncogene 2019. PMID 31616060.
3. Huang et al., Establishing an RNA fusions panel in soft tissue sarcoma with clinical validation. Scientific Reports 2023. PMID: 36928336.
4. Perry et al., Biology and Therapy of Dominant Fusion Oncoproteins Involving Transcription Factor and Chromatin Regulators in Sarcoma.
Annual Review of Cancer Biology, 2019. doi: 10.1146/annurev-cancerbio-030518-055710.
5. Mertens et al., Gene fusions in soft tissue tumors: Recurrent and overlapping pathogenetic themes. Genes Chromosomes Cancer, 2015.
PMID 26684580.
6. Dobin et al., STAR: ultrafast universal RNA-seq aligner. Bioinformatics 2013. PMID 23104886.
7. Haas et al., Accuracy assessment of fusion transcript detection via read-mapping and de novo fusion transcript assembly-based methods.
Genome Biology, 2019. PMID 31639029.
Accredited by DAkkS according to CLIA CERTIFIED ID: 99D2130225 DIN EN ISO 15189:2014
CeGaT is a global provider of genetic analyses for a wide range of
medical, research, and pharmaceutical applications.
Founded in 2009 in Tübingen, Germany, the company combines stateof-the-art sequencing technology with medical expertise – with the aim
of identifying the genetic causes of diseases and supporting patient
care. For researchers and pharmaceutical companies, CeGaT offers
a broad portfolio of sequencing services and tumor analyses. CeGaT
generates the data basis for clinical studies and medical innovations
and drives science forward with its own insights.
The owner-managed company stands for independence, comprehensive
personal customer service, and outstanding quality. CeGaT’s
laboratory is accredited according to CAP/CLIA, DIN EN ISO 15189, and
DIN EN ISO/IEC 17025 and thus meets the highest international
standards. To obtain first-class results, all processes are carried out
in-house under scientific supervision.
About Us
2023/08
CeGaT GmbH
Genetic Diagnostics
Paul-Ehrlich-Str. 23
72076 Tübingen
Germany
Phone: +49 7071 56544-220
Fax: +49 7071 56544-56
Email: diagnostic-support@cegat.com
Web: www.cegat.com/diagnostics
Brought to you by
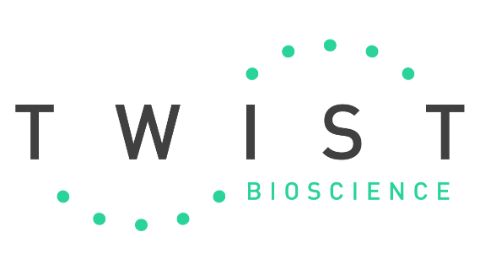
Download this App Note for FREE Below!
Information you provide will be shared with the sponsors for this content.
Technology Networks or its sponsors may contact you to offer you content or products based on your interest in this topic. You may opt-out at any time.
Experiencing issues viewing the form? Click here to access an alternate version