High-Throughput Analysis of PFAS in Air
App Note / Case Study
Published: July 2, 2024
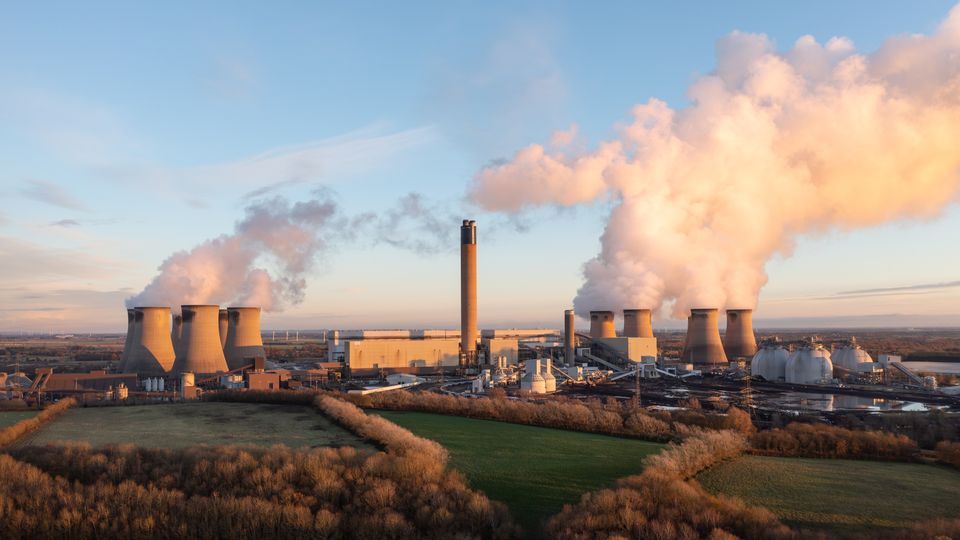
Credit: iStock
Airborne monitoring of per- and polyfluoroalkyl substances (PFAS) has become crucial in recent years due to their widespread environmental impact and ability to travel great distances in the atmosphere.
Cutting-edge methods such as thermal desorption coupled with gas chromatography mass spectrometry (TD-GC-MS/MS) allow for the simultaneous analysis of neutral (FTOH, FOSA) and ionic (PFCA, FTCA) PFAS in the air.
This application note showcases a fast, high-throughput approach to measure 19 target PFAS compounds with precision and efficiency.
Download this application note to discover:
- Advanced techniques for analyzing both neutral and ionic PFAS
- The benefits of thermal desorption for high-sensitivity detection
- Practical insights to improve analytical capabilities
Goal
The aim of this application note is to demonstrate a high-throughput method for the
simultaneous analysis of neutral (FTOH, FOSA) and ionic (PFCA, FTCA) per- and
polyfluoroalkyl substances (PFAS) in air using thermal desorption coupled to gas
chromatography mass spectrometry.
Introduction
Per- and polyfluoroalkyl substances (PFAS) contain one or more alkyl radicals with all the
hydrogens replaced by fluorine atoms. Traditionally, two groups of PFAS have been of the
most concern and subject for control and monitoring. The first group includes ionic (or
acidic) PFAS—the perfluorocarboxylic acids (perfluorooactanoic acid (PFOA) being the
most infamous one) and perfluoroalkylsulfonates (perfluorooctanesulfonic acid (PFOS), the
most infamous), where LC-MS-MS is the most commonly chosen analytical technique.
The second group includes neutral (or volatile) PFAS—the fluorotelomer alcohols1
(FTOHs)
and N-substituted fluoroalkylsulfonamides (FOSAs). For this group, GC-MS is the
analytical method of choice. Detecting airborne concentrations of both ionic and neutral
PFAS is critical for measuring atmospheric emission distribution and potential human
exposure. Unlike water and soil matrices, there are fewer factors that slow the dispersal of
PFAS after release into ambient air. These compounds can travel thousands of kilometers
from the original point of emission, which could be chemical manufacturing sites, thermal
waste treatment plants, or commercial applications of PFAS2
.
High-throughput analysis of both neutral and ionic PFAS
in ambient air using thermal desorption coupled to gas
chromatography – mass spectrometry (TD-GC-MS/MS)
Application note | 001715
Authors
Laura Miles,1
Hannah Calder,1
Vladimir
Nikiforov,2
Dorte Herzke,2
Nicholas Warner,3
Giulia Riccardino,4
Adam Ladak,5
and
Daniel Kutscher3
1
Markes International Ltd, Bridgend, UK
2
NILU-Norwegian Institute for Air Research,
Fram Centre, Tromsø, Norway
Thermo Fisher Scientific
3Bremen, DE; 4
Milan, IT;
5
Hemel Hempstead, UK
Keywords
Polyfluoroalkyl substances (PFAS),
air monitoring, pollution, thermal
desorption, TD 100-xr Advanced, gas
chromatography-mass spectrometry,
GC-MS, triple quadrupole, TSQ 9610,
Advanced Ionization Ion source (AEI),
TRACE 1610 GC
Environmental
Thermal desorption (TD) represents a suitable technique for
analysis of organic contaminants in air, including ultra-volatile
species. Stainless-steel tubes packed with special sorbents
are used as sampling media to preconcentrate samples from
hundreds of liters of air. This inherent preconcentration effect
with no requirement for any dilution prior to analysis means that
single-digit pg/m3
concentrations can be measured from <500 L
of ambient air when combined with triple quadrupole GC-MS/MS.
In this study, a single, fast, and high-throughput method for the
analysis of 19 target PFAS across four functional groups including
ionic perfluoroalkylcarboxylic acids (PFCAs) and fluorotelomer
carboxylic acids (FTCAs) and neutral FTOHs and FOSAs is
demonstrated for ambient air, using thermal desorption coupled
to gas chromatography mass spectrometry.
Experimental
In the experiments described here, a Markes International™
TD100-xr™ Advanced thermal desorber with electronic flow
control and equipped with the Internal Standard Addition / Dry
Purge (ISDP) accessory for automated addition of gaseous
internal standard was used. This sample introduction technique
was coupled to a Thermo Scientific™ TRACE™ 1610 GC
connected to a Thermo Scientific™ TSQ™ 9610 triple quadrupole
mass spectrometer, equipped with an Advanced Electron
Ionization (AEI) ion source. Chromatographic separation was
achieved on a Thermo Scientific™ TraceGOLD™ TG-200MS
capillary column (30 m × 0.25 mm × 1.0 μm, P/N 26084-2960).
The trifluoropropylmethyl polysiloxane stationary phase provides
exceptional inertness for improved thermal stability, low column
bleed, and reliable run-to-run and batch-to-batch reproducibility.
The Markes International TD100-xr thermal desorption unit
offers sequential and unattended analysis of up to 100 samples,
eliminating the cost of liquid cryogen through the use of
electrical trap cooling. Markes’ systems also allow samples to be
split and re-collected onto a clean sorbent tube at the sample
desorption and/or trap desorption stages, providing “insurance”
against failed runs and simplifying demonstration of complete
analyte transfer and absence of analytical bias. TD100-xr and
GC-MS/MS experimental conditions optimized in this study as
well as a complete list of the target compounds are detailed in
Table 1 and Table 2 (page 6), respectively.
Markes International TD100-xr Advanced parameters
Tube type PFAS Extended volume tubes
(P/N 76473-0942)
Flow path temperature (°C) 200
Automatic dry purge 1 min at 50 mL/min
Tube desorption 300 °C for 10 min at 50 mL/min
Trap purge 1 min at 50 mL/min
Focusing trap PFAS focusing trap
(P/N 76473-0940)
Focusing trap low temperature (°C) -30
Elevated trap purge (°C) 25
Focusing trap high temperature (°C) 300 (4 min)
Trap heat rate Max
Outlet split 6:1
TRACE 1610 GC parameters
Oven temperature program
Temperature (°C) 35
Hold time (min) 2
Rate (ºC/min) 15
Temperature 2 (°C) 280
Hold time (min) 5
GC run time (min) 23.33
Carrier gas He
Carrier flow (mL/min) 1.2
Column
TraceGOLD TG-200MS 30 m, 0.25 mm, 1.0 μm
(P/N 26084-2960)
TSQ 9610 mass spectrometer parameters
Transfer line temperature (°C) 280
Ion source type and temperature (°C) AEI, 300
Ionization type EI
Emission current (µA) 50
Aquisition mode timed-SRM
Tuning parameters AEI Smart Tune
Collision gas and pressure (psi) Argon at 70
Table 1. TD100-xr Advanced and GC-MS/MS experimental
conditions for the analysis of PFAS in air
2
Data acquisition, processing, and reporting
Data were acquired, processed, and reported using the Thermo
Scientific™ Chromeleon™ Chromatography Data System (CDS)
software, version 7.3. Integrated TD100-xr instrument control
ensures full automation of the analytical workflow from tube
desorption to data acquisition, combined with an intuitive user
interface for data analysis, processing, customizable reporting,
and storage in compliance with the Federal Drug Administration
Title 21 Code of Federal Regulations Part 11 (Title 21 CFR
Part 11). Figure 1 shows the Chromeleon CDS method editor
wizard for the TD100-xr thermal desorber.
Figure 1. Chromeleon CDS browser showing the method editor wizard for the TD100-xr thermal desorption unit: (A) 2-3 Stage
Desorb settings and (B) additional settings.
A
B
3
Standard and sample preparation
Standard preparation
Individual component standards were purchased from Wellington
Laboratories Inc, Canada, at a concentration of 50 ng/μL, with
exception for the PFCAs, which were available in a mixture at
2 ng/μL and used as a stock standard. The individual component
standards were combined and diluted to obtain the working
standards used to assess the method performance. A calibration
curve was prepared by using a Markes International™ Calibration
Solution Loading Rig (CSLR™) by spiking 1 μL of each standard
onto the sorbent tubes in a flow of nitrogen at 100 mL/min
and purged for 60 minutes to remove methanol. A Markes
International™ TC-20™ unit was used to purge up to 20 tubes
simultaneously, significantly speeding up the spiking process.
The TC-20 was also used to re-condition the sorbent tubes in
nitrogen prior to sampling, freeing up the analytical instrument
and saving helium.
Sample preparation
Ambient air from three sites at an industrial park were collected
onto a sorbent tube at a flow rate of 100 mL/min using a Markes
International™ ACTI-VOC PLUS™ constant flow sampling pump
for 50 hours until a volume of 300 L was reached. Flow rates for
sampling onto TD tubes typically range from 10 to 500 mL/min.
Sample flow rates can be optimized to enable sampling across
the desired time window, though higher flow rates may affect
breakthrough volumes. TD tubes can be used over 100 times
in their lifetime as the sorbent is re-generated each time it is
heated. Toluene-d8 gaseous internal standard at 100 ppb in
nitrogen was automatically added by using the ISDP accessory of
the TD100-xr and used to monitor the process to ensure sample
integrity. The IS (2 mL) was added to the sample tubes by using a
1 mL loop pressurized to 15 psi.
Results and discussion
Background assessment
One of the main challenges of PFAS analysis lies in the low
detection limits that must be achieved as these compounds
occur at trace concentrations in air. Dedicated PFAS tubes
packed with sorbent material (P/N 76473-0942) have been
designed to allow effective sample pre-concentration to
detect such low levels of PFAS. It is therefore vital that sorbent
tubes used for sampling are free from contamination. It is also
important to highlight that artifacts from the sorbent materials
may occur at very low levels upon heating, therefore the system
background and possible artifacts need to be assessed before
embarking on sampling projects. The artifacts contribution was
evaluated by desorbing the dedicated PFAS focusing trap of the
TD100-xr
(P/N 76473-0940) under the optimized method conditions
reported in Table 1. The instrument background was assessed
by desorbing an empty stainless-steel sorbent tube applying
the aforementioned optimized conditions. The method blank
was then evaluated by desorbing at least n=7 PFAS extended
range sorbent tubes and comparing the blank chromatograms
with the ones obtained during the method detection limit (MDL)
assessment.
No background was detected for the target species in the trap
and valve blank. In the system blank, only perfluorotetradecanoic
acid (PFTeDA) was detected at 9 pg versus the 5 pg spiked
level used to assess the MDL. For context, during the sampling
study, the mass of PFTeDA collected (when present in the
sample) was five to 10 times higher. For the method blank, five
compounds were found to be above the spike level. One of these
compounds was not present in all the tubes tested, indicating
the importance of not just looking at the average blank value
4
Figure 2. Mixed PFAS standard at 500 pg on-tube. The inset shows a close-up view of the chromatogram for the first five compounds, which are
perfluoroalkylcarboxylic acids (PFCAs). Baseline separation was not possible for PFBA and PFPeA at the oven start temperature of 35 °C.
but also understanding variations with a view to choosing
tubes for field sampling that do not show background for target
compounds. The calculated method detection limit (MDL) for
these compounds reflects the level at which they were found in
the method blank.
Chromatography
PFAS are a group of synthetic compounds with different
chemical and physical properties, therefore the proper column
choice is essential to get adequate resolution and sensitivity
for both qualitative and quantitative analysis. The polar phase
of the TraceGOLD TG-200MS column provided adequate
chromatographic separation of the target compounds combined
with Gaussian peak shapes as demonstrated in Figure 2.
1.36 1.60 1.80 2.00 2.20 2.40
0.0e0
5.0e4
1.0e5
1.5e5
2.0e5
2.5e5
3.0e5
3.5e5
4.0e5
4.5e5
5.0e5
5.5e5
6.0e5
PFPeA
PFHxA
PFHpA
PFO
A
PFBA
1 2 3 4 5 6 7 8 9 10 11 12 13 14
0.0e0
1.0e5
2.0e5
3.0e5
4.0e5
5.0e5
6.0e5
7.0e5
8.0e5
9.0e5
1.0e6
1.1e6
1.2e6
1.3e6
1.4e6
1.5e6
1.6e6
1.7e6
PFPe
A
PFHxA
PFHp
A
PFOA
PFNA
PFDA
FHEA
PFUd
A
PFDoA Toluene D8 IS
FOE
A
FBET PFTrDA
PFTeDA
FHET
FOE
T
FDET
Me-FOSA
Et-FOSA
Intensity [counts]
Time [min]
PFBA
5
Table 2. List of target compounds as well as retention times (RT), quantitation ions, coefficient of determination (R2), peak area %RSD (n=7)
and calculated MDLs.
Compound
RT
(min)
Quantitation
ion
(m/z)
Calibration
range
(pg/µL) R2
Peak area
%RSD
(n=7)
Calculated
MDL
(pg)
Calculated
MDL
(pg/m3
)
Perfluoroalkyl carboxylic acids (PFCAs)
Perfluoro-n-butanoic acid (PFBA) 1.59 131/69 10-2000 0.9985 4.52 5 10
Perfluoro-n-pentanoic acid (PFPeA) 1.64 131/69 10-2000 0.9966 3.8 2 4
Perfluoro-n-hexanoic acid (PFHxA) 1.73 131/69 10-2000 0.9970 3.25 23 46
Perfluoro-n-heptanoic acid (PFHpA) 1.93 131/69 10-2000 0.9981 2.42 3 6
Perfluoro-n-octanoic acid (PFOA) 2.31 131/69 10-2000 0.9986 2 2 4
Perfluoro-n-nonanoic acid (PFNA) 2.9 131/69 10-2000 0.9983 1.48 46 95
Perfluoro-n-decanoic acid (PFDA) 3.67 131/69 10-2000 0.9978 2.48 27 54
Perfluoro-n-undecanoic acid (PFUdA) 4.52 131/69 10-2000 0.9974 3.67 4 8
Perfluoro-n-dodecanoic acid (PFDoA) 5.39 131/69 10-2000 0.9975 2.71 21 42
Perfluoro-n-tridecanoic acid (PFTrDA) 6.22 131/69 10-2000 0.9974 3 3 6
Perfluoro-n-tetradecanoic acid (PFTeDA) 6.98 131/69 10-2000 0.9975 3.01 2 4
Fluorotelomer carboxylic acids (FTCAs)
2-Perfluorohexyl ethanoic acid (6:2) (FHEA) 3.97 131/69 100-5000 0.9953 5.75 64 128
2-Perfluorooctyl ethanoic acid (8:2) (FOEA) 5.9 131/69 100-5000 0.9983 2.65 62 104
Fluorotelomer alcohols (FTOHs)
2-Perfluorobutyl ethanol (4:2) (FBET) 6.01 95/69 10-5000 0.9951 4.1 13 26
2-Perfluorohexyl ethanol (6:2) (FHET) 7.67 95/69 10-5000 0.9971 2.61 18 36
2-Perfluorooctyl ethanol (8:2) (FOET) 9.12 95/69 10-5000 0.9963 3.99 4 8
2-Perfluorodecyl ethanol (10:2) (FDET) 10.41 95/69 10-5000 0.9937 4.08 6 12
Perfluorooctanesulfonamides (FOSAs)
N-Methylperfluoro-1-octanesulfonamide Me-(FOSA) 12.87 94/30 10-5000 0.9953 0.83 1 2
N-Ethylperfluoro-1-octanesulfonamide Et-(FOSA) 13.18 108/80 10-5000 0.9953 5.29 1 2
Linearity
Calibration curves were prepared by spiking 1 µL of the stock
solution on the sorbent tubes. Due to the concentrations of the
stock standards, different compound classes were calibrated
over different ranges. All compounds showed a linear trend within
the used calibration ranges with the coefficient of determination
(R2
) > 0.990 as reported in Table 2.
Method detection limits
The concentration of individual PFAS species in ambient air varies
depending on location, i.e., urban versus rural environments.
Background monitoring sites, usually in remote locations such as
mountains, heavy forests or the Polar Regions, typically report
PFAS concentrations of <1 and up to 200 pg/m3.
4
In urban
environments, due to the presence of multiple PFAS sources,
typical concentrations can be much higher (up to 800 pg/m3),
depending on the compound.4
The MDL was calculated by comparing n=7 method blanks
with n=7 sorbent tubes that were spiked with a standard at a
“challenge level” in accordance with U.S. EPA guidance.5
As
described earlier, this process was designed to account for any
background contaminants introduced during sample handling,
preparation, and analysis. The average limit of detection was
9 pg on tube corresponding to 31.2 pg/m3
when considering the
sampled air volume as reported in Table 2. These values match
the lower concentration range for both background and urban
monitoring sites.
Analysis of ambient air samples
Ambient air from three sites (Sites 1, 2, and 3) within the industrial
park sampling site were collected at a volume of 300 L. When
sampling such a large air volume, a significant amount of water
vapor from the air is trapped on the sorbent tubes together with
the analytes. Moisture must be removed prior to injecting the
samples into the gas chromatograph as this can negatively affect
the column performance, reducing reproducibility of the analysis.
The TD100-xr offers an integrated water management system
that combines a dry purge of the tubes before desorption with
an elevated temperature trap purge. This allows for the removal
of most of the water vapor from the sorbent tubes and the
focusing trap prior to injection while retaining 100% of the target
analytes. After sampling, the tubes were stored until analysis.
6
Figure 3. XIC ambient air sampled in a light industrial location (A) as well as SRM transitions for some of the detected compounds (B).
Compounds were identified from each of our target classes (PFCAs, FTOHs, FTCAs, FOSAs) with PFCAs being the most abundant.
Each sample tube was analyzed twice using the automated
re-collection feature of the TD100-xr, offering the possibility of
analyzing the samples for both targeted analysis by using an
optimized SRM method and untargeted screening of other volatile
compounds by using full-scan acquisition (m/z 35–650). Most
of the compounds monitored were found at each site, with the
exception of PFHpA, PFUdA, FBET, PFTrDA, FDET, and EtFOSA.
The compounds with the highest concentrations were carboxylic
acids (PFBA, PFHxA, and PFOA) as reported in the Appendix.
In many studies, PFOA is often the polyfluorinated carboxylic
acid with the highest concentration, so it is interesting that PFBA
was detected at a higher level here. An example of extracted ion
chromatogram (XIC) for air samples in site 1 (A) as well as SRM
transitions for some of the detected compounds (B) are shown in
Figure 3.
1.2 1.5 2.0 2.5 3.0 3.5 4.0 4.5 5.0 5.5 6.0 6.5 7.0 7.5 8.0 8.5 9.0 9.5 10.0 10.5
0.0e0
5.0e4
1.0e5
1.5e5
2.0e5
2.5e5
3.0e5
3.5e5
4.0e5
4.5e5
5.0e5
5.5e5
6.0e5
6.5e5
7.0e5
7.6e5
Intensity [counts]
Time [min]
PFHxA
PFHp
A
PFOA
PFNA
PFD
A
PFBA
FHEA
PFDoA
Toluene D8 IS
FBET
PFTeDA
FHET
FOET
FDET
A
PFOA PFNA FOET FDET B
7
Conclusions
The results obtained in these experiments demonstrate that
the TD100-xr Advanced coupled to the TRACE 1610 GC and
TSQ 9610 mass spectrometer equipped with the AEI ion source
delivers reliable analytical performance for analysis of PFAS in air,
allowing for the analysis of the volatile neutral PFAS species and
volatile ionic PFCAs in a single run.
• Adequate chromatographic separation was achieved for the
investigated compounds in <14 minutes thanks to the high
selectivity of the TraceGOLD TG-200MS column, allowing for
high sample throughput analysis.
• Accurate quantitative performance for the investigated
compounds was obtained with coefficient of determination of
R2
> 0.990 over a concentration range of 10 to 5,000 pg/µL
(compound dependently).
• Low detection limits were achieved with average MDL of
31.2 pg/m3
calculated considering a sample volume of 300 L.
• Most of the investigated compounds were found in the
analyzed air samples with carboxylic acids (PFBA, PFHxA,
and PFOA) being present with the highest concentrations.
• Targeted analysis and untargeted screening of the same
sample are possible thanks to the sample re-collection
capability of the Markes' TD systems.
References
1. Ellis D.A.; Martin J.W.; Mabury S.A.; Hurley M.D.; Sulbaek Andersen M.P.; Wallington
T.J. Atmospheric Lifetime of Fluorotelomer Alcohols, Environmental Science &
Technology, 2003, 37, 3816–3820.
2. Interstate Technology & Regulatory Council (ITRC), updated 2020. PFAS Technical and
Regulatory Guidance Document and Fact Sheets PFAS-1. Washington, D.C.: Interstate
Technology & Regulatory Council, PFAS Team. PFAS_Fact_Sheet_History_and_Use_
April 2020.pdf, https://itrcweb.org/home
3. D’Ambro E.L.; Pye H.O.T.; Bash J.O.; Bowyer J.; Allen C.; Efstathiou C.; Gilliam R.C.;
Reynolds L.; Talgo K.; Murphy B.N. Characterizing the Air Emissions, Transport, and
Deposition of Per- and Polyfluoroalkyl Substances from a Fluoropolymer Manufacturing
Facility, Environmental Science & Technology, 2021, 55. 862–870.
4. Rauert C.; Shoieb M.; Schuster J.K.; Eng A.; Harner T. Atmospheric Concentrations and
Trends of Poly- and Perfluoroalkyl Substances (PFAS) and Volatile Methyl Siloxanes
(VMS) over 7 Years of Sampling in the Global Atmospheric Passive Sampling (GAPS)
network, Environmental Pollution, 2018, 238, 94–102.
5. U. S. Environmental Protection Agency (EPA). (2016a). Definition and Procedure for
the Determination of the Method Detection Limit, Revision 2. U.S. EPA Office of Water,
EPA 821-R-16-006, https://www.epa.gov/sites/default/files/2016-12/documents/
mdl-procedure_rev2_12-13-2016.pdf
8
General Laboratory Equipment – Not For Diagnostic Procedures. ©2023 Thermo Fisher Scientific Inc. All
rights reserved. Markes International, TD100-xr, Calibration Solution Loading Rig, CSLR, TC-20, and ACTI-VOC PLUS are trademarks
of Markes International. All other trademarks are the property of Thermo Fisher Scientific and its subsidiaries. This information is
presented as an example of the capabilities of Thermo Fisher Scientific products. It is not intended to encourage use of these products
in any manners that might infringe the intellectual property rights of others. Specifications, terms, and pricing are subject to change.
Not all products are available in all countries. Please consult your local sales representative for details. AN001715-EN 0123S
Learn more at thermofisher.com
Appendix
Table A1. Calculated amounts for the target PFAS compounds found at each site in the light industrial
area
*Compounds detected below the MDL.
Amount (pg/m3
)
Compound RT (min) Site 1 Site 2 Site 3
Perfluoroalkyl carboxylic acids (PFCAs)
Perfluoro-n-butanoic acid (PFBA) 1.59 2903 2097 4790
Perfluoro-n-pentanoic acid (PFPeA) 1.64 ND ND ND
Perfluoro-n-hexanoic acid (PFHxA) 1.73 850 750 1000
Perfluoro-n-heptanoic acid (PFHpA) 1.93 ND 403 477
Perfluoro-n-octanoic acid (PFOA) 2.31 1267 1433 3090
Perfluoro-n-nonanoic acid (PFNA) 2.9 *60 217 520
Perfluoro-n-decanoic acid (PFDA) 3.67 147 *17 103
Perfluoro-n-undecanoic acid (PFUdA) 4.52 23 ND ND
Perfluoro-n-dodecanoic acid (PFDoA) 5.39 333 *33 287
Perfluoro-n-tridecanoic acid (PFTrDA) 6.22 27 ND ND
Perfluoro-n-tetradecanoic acid (PFTeDA) 6.98 170 ND ND
Fluorotelomer carboxylic acids (FTCAs)
2-Perfluorohexyl ethanoic acid (6:2) (FHEA) 3.97 ND ND ND
2-Perfluorooctyl ethanoic acid (8:2) (FOEA) 5.9 ND ND ND
Fluorotelomer alcohols (FTOHs)
2-Perfluorobutyl ethanol (4:2) (FBET) 6.01 ND ND ND
2-Perfluorohexyl ethanol (6:2) (FHET) 7.67 ND ND ND
2-Perfluorooctyl ethanol (8:2) (FOET) 9.12 ND ND *3
2-Perfluorodecyl ethanol (10:2) (FDET) 10.41 137 ND ND
Perfluorooctanesulfonamides (FOSAs)
N-Methylperfluoro-1-octanesulfonamide Me-(FOSA) 12.87 130 107 113
N-Ethylperfluoro-1-octanesulfonamide Et-(FOSA) 13.18 180 ND ND
Brought to you by
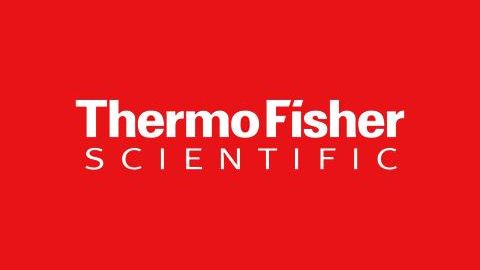
Download this App Note for FREE Below!
Information you provide will be shared with the sponsors for this content.
Technology Networks or its sponsors may contact you to offer you content or products based on your interest in this topic. You may opt-out at any time.
Experiencing issues viewing the form? Click here to access an alternate version