Enhancing Immune Cell Cytotoxicity and Persistence Insights Using Real-Time Cell Analysis
Whitepaper
Published: February 21, 2024
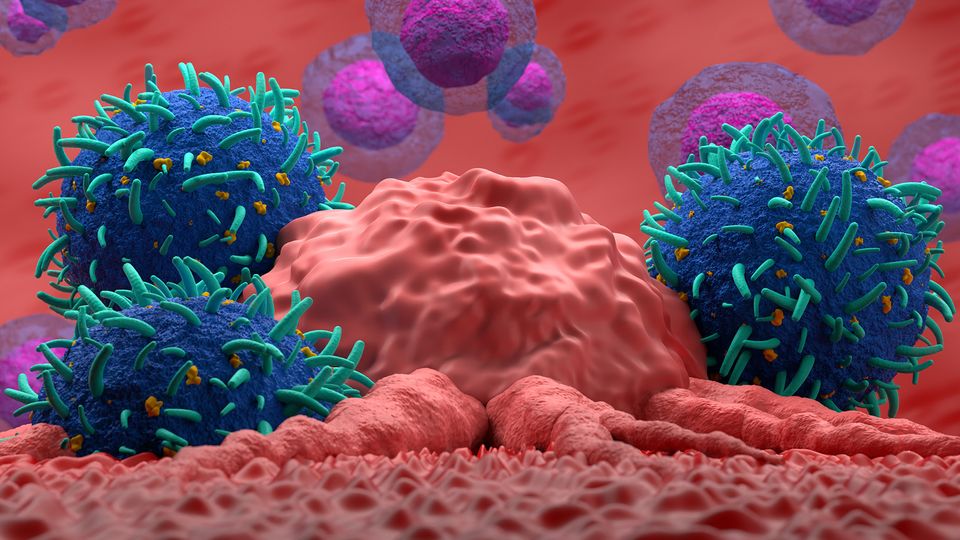
Credit: iStock
Cell therapies, from regenerative medicine to immunotherapy, are transforming the medical landscape with 75 already approved globally and over 2,000 in development.
As the field grows, there is a need for robust and reproducible potency assays to capture the full spectrum of effector cell activities, throughout process development and manufacturing, to ensure the safety of the end product.
Explore how cellular impedance assays using the latest real-time cell analysis (RTCA) platform can overcome the limitations of traditional endpoint assays in immunotherapy development, provide label-free, rapid and highly sensitive insights into cell potency, and support the requirements of 21 CFR Part 11.
Download this article to discover:
- Challenges faced in measuring immune cell cytotoxicity
- Potency assays available for cellular immunotherapies and the regulatory criteria they must meet
- An real-time cell analysis platform that can facilitate significant time and cost savings
Enhancing Immune Cell
Cytotoxicity and Persistence
Insights Using Real-Time Cell
Analysis
Article
Introduction
Cell therapies – the transfer of autologous or allogenic cells for therapeutic
means – have evolved into a highly promising area of drug development
for a wide range of applications, including regenerative medicine and
immunotherapy. As of 2023, 65 non-genetically modified cell therapies, and 10
genetically modified cell therapies have already been approved globally and
are available for clinical use.1 With more than 2,000 further therapies currently
in development, cell therapy has the potential to dramatically transform the
medical landscape, providing treatments and reducing patient suffering.
As advanced therapy medicinal products (ATMPs), cell therapies are
required to meet defined safety, purity, and potency requirements as part of
their process development and approval criteria. In order to meet potency
requirements, cell therapies must demonstrate the ability to safely exert a
defined biological effect.2,3 However, as cell therapeutics are “living drugs”
consisting of live effector cells, the mechanism of action (MoA) can be
complex, multi-faceted, and difficult to determine using one single assay or
marker.4 As a result, potency assays must be robust and reliable, and capture
the full spectrum of effector cell activities.
This editorial will discuss the potency assays available for cellular
immunotherapies, the regulatory criteria they must meet, and the challenges
faced in measuring immune cell cytotoxicity. It will also explore the solutions
available to overcome these challenges and how real-time cell analysis
platforms are advancing the field of immunotherapy.
2
Optimizing the development of cellular
therapies
Since the approval of the chimeric antigen receptor (CAR)
T-cell therapy tisagenlecleucel (Kymriah) to treat B-cell acute
lymphoblastic leukemia in 2017, immune cell-based adoptive
cell therapies (ACTs), have seen extensive progress.5 CAR
T-cell therapies are often autologous, using the patient’s
own genetically edited immune cells to circumvent the
immunosuppressive properties of tumors and destroy cancer
cells.6 There are currently six CAR T-cell therapies approved for
clinical use in the United States, all of which are produced by a
similar method (Figure 1).
Although CAR T-cell therapy has been highly successful
in treating hematological malignancies, there has been
limited success in CAR T-cell therapies for solid tumors.7,8
Efforts can be inhibited by a lack of tumor-specific antigens,
limited infiltration into solid tumor tissue, and the highly
immunosuppressive tumor microenvironment (TME).
In addition, the need for exact major histocompatibility
complex (MHC)-matched donor cells can limit harvesting
potential and delay treatment.5 CAR T-cell therapies can also
be associated with cytokine release syndrome (CRS) – a
severe toxicity event induced by the powerful CAR T celldirected
immune response. Although this can be managed
with immunosuppressants, it can be a considerable concern
for recipients.9 To overcome these limitations, CAR natural
killer (NK) and macrophage cell therapies are currently in
development. CAR NK cells can directly recognize tumor
antigens for a rapid cytotoxic response, without the need for
MHC antigen presentation.5 The less specific nature of the
NK cell response limits graft versus host disease in allogenic
settings, and increases the feasibility of “off-the-shelf”
manufacturing at a fraction of the price of CAR T cells.10 CRS
can also be avoided in CAR NK-cell therapies, as the NK cell
cytokine release profile is less inflammatory than that of T
cells.11 Pre-clinical studies of CAR macrophage therapies have
shown improved infiltration of solid tumors, boosting of antitumor
T cell responses, and significantly improved survival in
mice.12
In recent years, cellular bioenergetics has been shown to
be key in understanding the fate, function, and fitness of
immune cells, including genetically modified T cells. The
metabolic poise of immune cells is a critical determinant
that is intrinsically linked to anti-tumor efficacy, especially
within the immunosuppressive tumor microenvironment.13,14
❺ CAR T cells
attack cancer cells ❹ Infuse CAR T
cells into patient
❶ Acquire T cells from a blood sample
❷ Engineer
CAR T cells
CAR T cell
Insert sequences
T cell for CAR
Death of
cancer
cells
Antigen-recognition
domain
Signaling
domains
❸ Expand
CAR T cells
Figure 1. Steps involved in producing CAR T-cell therapies. T lymphocytes are harvested and purified from the patient or donor’s blood. The T cells
are then activated, either by antigen-presenting cells also purified from the patient, or anti-CD3 antibodies coupled with growth factors such as
interleukin-2.15 CARs designed to target specific tumor antigens are integrated into the genomes of the activated T cells via delivery methods such
as mRNA transfection and lentiviral transduction.15 Once the CAR T-cell populations are expanded and infused back into the patient, the genetically
modified CARs are able to specifically recognize tumor cells, and destroy them using classic cytotoxic T lymphocyte methods such as the release of
perforin and granzymes.16
3
Therefore, the analysis and optimization of key metabolic
and bioenergetic parameters of immune cells are of critical
importance, both for understanding the biology of the antitumor
immune response and for therapeutic design.
Despite the limitations and ongoing improvement of CAR cell
therapies, the overall impact has been incredibly beneficial.
Clinical studies show high efficacy and increased survival
and remission rates, supported by the persistence of CAR T
cells.17,18 However, as a form of “living drug”, the complexity
and inherent heterogeneity of these therapies can result in
challenging regulatory processes. This heterogeneity generates
difficulty in bringing products to market and delays further
benefits to patients. Therefore, robust, reliable, and easily
scaled manufacturing and quality control processes are
essential.
The criteria for potency testing
As part of their complex approvals process, ACTs are
required to demonstrate consistent and reliable potency.
Regulatory bodies such as the United States Food and Drug
Administration (FDA) and the European Union’s European
Medicines Agency (EMA), provide guidance on the structure
and data requirements of potency assays, as well as current
good manufacturing practice (cGMP) guidelines to produce
approved cell therapies.3,19 The exact requirements vary
between regulating bodies, but the overall purpose of a
potency assay is consistent. These assays must determine
the specific ability of the therapeutic to effect the defined
MoA, as assessed by appropriate and rigorous clinical data or
laboratory testing. 3 To this end, potency assays are expected
to fulfill certain criteria. For example, recent draft guidance
published by the FDA highlighted the desirable characteristics
of potency assays, including: mitigation of risk to product
potency, precision, accuracy, specificity, robustness,
minimization of assay redundancy, and minimization the use
of animals. (Figure 2).20
In order to develop a potency assay for a cell therapy, the
MoA must first be characterized. However, as cell therapies
involve complex, living cells, these MoAs are also likely
to be complex and multifactorial. Cell products are also
inherently variable and sensitive to their environment.
Processing and storage can affect biological activity and
cell properties, with potentially significant effects on safety
or function. As a result, multiple potency assays (known as
an “assay matrix”) may be required to fully characterize the
biological mechanisms at all stages of process development,
manufacture, and release.21,22 Where possible, these assays
should comprise a functional evaluation of the product’s MoA,
such as a tumor cell killing assay for an ACT. However, if a
functional assay is unsuitable or unavailable, measurement of
a surrogate marker indicating biological activity can be used.2
Once the MoA is defined and a relevant and robust assay is
Figure 2. Potency assay requirements and challenges.
Predictive of Clinical Efficacy Meet Release Criteria
Representative of Mechanism
of Action Ensure Batch-to-Batch Consistency
Rapid Kinetics Sensitive and Specific
Covering All Product Constituents Accurate
Potency
Assays
4
selected, assay validation is performed and critical quality
attributes (CQAs) are identified. Appropriate reference
standards and controls must be selected, along with
predefined pass/fail release criteria.23 It is a mandatory
requirement that potency testing be carried out throughout
the process development and during manufacturing to ensure
batch consistency, product stability, and efficacy (Figure
3).3,24,25 Clinical efficacy and safety of cell therapies are closely
linked to the potency of engineered immune cells, and even
small deviations in potency may result in lack of effect or
adverse events in highly sensitive patients.4 Clinical studies
can be relatively insensitive to minor changes in potency
due to patient variability, therefore potency testing must also
continue throughout commercial production. These potency
assays must be tolerant of the inherent heterogeneity of
cellular products but also sensitive to meaningful changes in
potency to ensure consistency and safety. 3,26,27
The challenges of developing robust
potency assays
As cellular immunotherapies are so unique, appropriate
potency assays can vary significantly from product to
product. It is essential that these potency assays capture
the dynamic interactions between the therapeutic and
the target cell. For CAR products, potency assays are
predominantly cytotoxicity-based, aligning with the main
mechanism of action of both T cells and NK cells. In some
cases, surrogate markers of cytotoxic activity are employed,
such as the induction of degranulation markers (CD107a or
granzyme B), or of inflammatory and pro-apoptotic cytokines
(most commonly IFN-γ, TNFα or IL-2).28 The four most
common in vitro cytotoxicity assays are cellular impedance,
bioluminescence, and flow cytometry. The chromium release
assay has, in the past, been considered the standard of
functional potency testing for cellular immunotherapies. In
this assay, target cells are labeled with radioactive chromium
(51Cr) and incubated with the activated CAR cells. Following
effector-mediated killing, 51Cr is released into the culture
medium, proportionally to the number of cells destroyed.29
Other cytotoxicity assays include the bioluminescence
luciferase assay (in which cytotoxicity is assessed by the
relative reduction in luminescence emitted by target cells
expressing a luciferase transgene) and flow cytometry
(whereby specific target cell populations are enumerated
using fluorescently-labeled antibodies).30,31
Label-based assays, such as chromium release and
bioluminescence assays, rely on target cells stably expressing
a transgene, or being labeled by the radioactive isotope. This
Figure 3. Significance of potency assays for cell therapies during product
development.
Potency
Assay
Potency
Assay
Potency
Assay
Clinical Use
✔ Ensure intended clinical effect
✔ Continued assurance of
efficacy and safety
✔ Monitor and optimize dosage
Manufacturing and Release
✔ Meet specifications
✔ Ensure batch comparability
✔ Identify subpotent batches
✔ Monitor product stability
Development and
Characterization
Tools: ELISA, qPCR, PCR, Flow
Cytometry, HPLC, TCID50
✔ Exert intended biological effect
✔ Correlation between product
constituents and activity
✔ Demonstration of other key features
of biological activity
Tools: ELISA, qPCR, PCR, Flow
Cytometry, HPLC, TCID50
5
can be time- and labor-consuming and is not necessarily
suitable for every type of cell. Moreover, the nature of these
label-based assays requires multiple manual procedures,
which can easily increase variability. In addition, the use of
radioactivity in the chromium release assay requires a level
of infrastructure and licensing that not all laboratories may
possess, the radioactivity involved can be harmful to lab
personnel, and cells can leak chromium non-specifically.
Despite the range of potency tests available for cell therapies,
numerous challenges remain in developing robust, accurate
assays with a rapid turnaround. The autologous nature of
many cell therapies such as CAR T cells poses multiple
challenges in potency assay development. Limited availability
of cells for testing means that assays must be developed to
use as little material as possible. In addition, the high levels of
heterogeneity between patients make quantification of CQAs
difficult due to a lack of standardized reference material.23
Several of these common cytotoxicity assays, such as the
chromium release assay and bioluminescence assay, are
endpoint in nature. Therefore, complex kinetic data modeling
of the dynamic interactions of target and effector cells can
❶ Cell Adhesion
When cells have been added
to the wells, there is a rapid
increase in impedance via
cell adhesion and attachment
❷ Cell proliferation
Cells begin to undergo
proliferation and
increase in number
❸ Cellular confluency
or barrier formation
When cells reach
confluency, the Cell Index
(CI) value plateaus
❹ Effector cell
addition
Addition of immune
effector cells causes
CI to decrease.
❺ Cell death,
shrinkage or
detachment
Cells begin to round
and detach from
the well bottom, as
they undergo death.
Cell index
Time (hours)
Culture
medium
Gold
biosensors
Cells
Electron
flow
Electron flow through culture media Electron flows are impeded by adherent cells
Figure 4. The mode of action of a cellular impedance assay.
6
be lost, as can information on effector cell persistence. Rates
and efficiency of effector cell killing can vary over the course
of the experiment, making endpoint dependent assays less
reliable than real-time analysis, as endpoint assays can only
provide a single value. In addition, these assays can be timeconsuming
with complex setups, effectively restricting their
throughput capabilities and their utility for certain autologous
therapies that require rapid turnarounds.
While other potency assay options can surmount some of the
challenges of complex endpoint assays, they too have their
own limitations. For example, flow cytometry assays offer
both direct measurement of potency (e.g., measuring CD19
levels as a direct evaluation of malignant B cell killing by CAR
T cells) and the simultaneous analysis of various surrogate
markers (e.g., CD107a as a marker of cytotoxic T cell
degranulation).32,33 However, some studies suggest that these
Adherent target cell (such as
tumor cells) are seeded into
the xCELLigence Biosensor
E-Plate wells. As cells
proliferate and attach to the
biosensors, the Cell Index
(CI) increases.
Non-adherent effector cells
(such as CAR T cells) are
added to the wells. The CI
remains unchanged upon
this addition.
The CI will decrease if the
effector cells kill the tumor
cells. Thus, the cell therapy’s
potency is sensitively and
precisely detected using
the accompanying GMP
compliant software.
STEP 3 STEP 2 STEP 1
Cell index Cell index Cell index
Time (h)
Proliferation
Add target
cells
+ Effector
cells
+ Effector
cells
Cytolysis
0:1 (control)
Effector:
Target
ratio
Cell index decreases as target cells are killed
0.1:1
0.5:1
1:1
Time (h)
Time (h)
Figure 5. The xCELLigence RCTA workflow utilizes impedance to monitor cell killing.
7
surrogate markers of in vitro cytotoxicity do not necessarily
show a significant correlation with clinical efficacy.34 In vivo
models of potency can offer a more accurate picture of
clinical efficacy, but animal studies are lengthy, expensive, and
require large numbers of animals, again limiting their utility for
potency assay development. With many of the conventional
potency assay options for cellular immunotherapies beset by
multiple challenges and limitations, there is a clear need for a
label-free, non-invasive, and sensitive solution that is able to
produce results in real-time.
The Real-Time Cell Analysis solution
Cellular impedance assays have gained traction in recent
decades as a label-free, non-radioactive alternative. This
assay measures the flow of an electric current between
microelectrodes embedded in the base of a biosensor
plate (Figure 4). Impedance of this current is correlated
with the number of cells in the well, in addition to their
viability, adhesion, and morphological characteristics.35,36
Cell impedance is measured in real time as “cell index” (CI),
a unitless scale that changes in proportion to the number of
cells over time.37
These real-time cellular assays are increasingly being used
to determine potency in cellular immunotherapeutics. They
can overcome the challenges raised by other popular potency
methods. Cellular impedance assays do not require radiation
and are both label-free and non-invasive. The real-time element
allows the analysis of both cytotoxicity and cellular persistence.
In addition, when compared directly to chromium release
assays, cellular impedance methods show increased sensitivity
to cellular lysis, making them an ideal solution for the potency
testing of cellular immunotherapies such as ACTs.38,39
The Agilent xCELLigence Real-Time Cell Analysis (RTCA)
platform offers a robust, simple, and automated solution for
cellular potency assays. The system measures quantitative
real-time kinetics with extremely high sensitivity and allows
for the study of diverse populations of effector and target
cells. xCELLigence RTCA technology can monitor cell number,
size, morphology, and attachment through cellular impedance
in both single plate and high-throughput scenarios. The ability
to house these instruments inside standard tissue culture
incubators or hypoxia chambers, enables fine-tuned control of
environmental conditions for live cell analysis. In addition, the
combination of cellular impedance with live cell imaging in the
xCELLigence RTCA eSight allows for increased confidence
in results and comprehensive insight into cellular health and
potency.
Each well of the specialized xCELLigence Biosensor E-Plates
is coated with gold biosensors that form an interdigitating
array covering approximately 70-80% of the surface of
the wells. This allows for large populations of cells to be
measured simultaneously, with top-notch sensitivity. For
measuring potency of cellular immunotherapies such as
CAR T cells, the workflow is more convenient and simpler
than other label-based assays (Figure 5). Adherent target
cells (i.e., tumor cells) are cultured in the E-Plate wells,
and the CI increases as they proliferate. As confluence
approaches 100%, non-adherent effector cells (e.g., CAR T
cells) are added. Immune effector cells are non-adherent
and therefore typically produce a minimal impedance signal.
Cytotoxic action by the effector cells will decrease CI, which
can be evaluated in real time with cGMP and 21CFR Part
11-compliant software, enabling an accurate, immediate
assessment of therapeutic potency.
xCELLigence RTCA solutions are already being used
extensively for cancer research around the globe, with proven
effectiveness in a variety of immunotherapy applications,
including CAR cell therapeutics, antibody-mediated
cytotoxicity, and oncolytic viruses.40 xCELLigence impedance
assays for CAR T cell potency against liquid cancers
have been tested and validated, confirming their potential
applications for this type of immunotherapeutic.41 Nonadherent
liquid tumor cells (e.g., leukemic cancer cells) stay
in suspension and thus require the use of a tethering kit for
impedence readout. Agilent’s xCELLigence Immunotherapy
Kits provide a tethering reagent (for example anti-CD29 or
anti-CD19) to enable non-adherent liquid cancer cells to be
immobilized for the assay.
Development of CAR T therapies against solid tumors has
also benefited from xCELLigence technology, as the RTCA
technology has been used to validate the potency of advanced
CAR T cells against mesothelioma and pancreatic cancer
models.42 In addition, these assays have also shown that
CAR T cell cytotoxicity against solid tumors is unaffected by
cryopreservation, which is key in developing therapies that
can be stably stored.43 In the developing field of CAR NK cells,
xCELLigence-based potency assays have proven the cytotoxic
efficacy of genetically modified NK cells and shown the ability of
CAR NK cells to work synergistically with current therapies.44,45
The benefits of xCELLigence impedance assays are not
limited to cellular cancer therapies. Investigation of potency
assays for monoclonal antibody immunotherapeutics have
shown that impedance-based techniques outperformed
standard release and membrane permeability assays, with
far more sensitive and accurate results.46 In addition, the
2022 “Recent Issues in Bioanalysis” workshop with thought
leaders in industry and regulatory bodies, highlighted
8
the xCELLigence RTCA system as the optimum moveforward
platform for characterization and clinical testing of
oncolytic viruses, over the traditional plaque assay.47 On the
regulatory compliance side, xCELLigence RTCA Software
Pro also supports the requirements of FDA 21 CFR Part 11
to ensure the authenticity and integrity of electronic data.
These high levels of data security protect the confidentiality
of research information, and aid in the generation of highly
accurate, reliable results, which are essential to progress
through the pre-clinical and clinical stages of biotherapeutic
developement.
Conclusion
As the field of cellular immunotherapies continues to
develop, the need for robust and reproducible potency
assays becomes increasingly urgent. For immunotherapies,
cellular impedance assays using the Agilent xCELLigence
RTCA platform can overcome the limitations of traditional
endpoint assays and provide real-time, label-free, rapid,
and highly sensitive insights into cell potency, and thus cell
killing. As such, the xCELLigence RTCA system can help
advance the development and manufacturing of various
cellular immunotherapeutics. Lastly, it can ensure compliance
with regulatory guidelines, facilitate significant time and
cost savings, and thus support the development of novel
therapeutics.
Learn more about
xCELLigence cell analyzers
Learn more about how
immune cell metabolism
is changing the immunooncology
discovery game
References
1. American Society of Gene & Cell Therapy, Citeline. Gene, Cell
and RNA Therapy Landscape Report Q3 2023 Quarterly Data
Report. American Society of Cell and Gene Therapy. https://
asgct.org/publications/news/october-2023/read-asgct-s-
2023-q3-landscape-analysis-field-repo. Published October 18,
2023. Accessed December 13, 2023.
2. Lowdell MW, Weil B. Bringing function to the forefront of cell
therapy: How do we demonstrate potency? Front Immunol.
2023;14. doi:10.3389/fimmu.2023.1226841
3. Food and Drug Administration. Guidance for Industry: Potency
Test for Cellular and Gene Therapy Products. https://www.fda.
gov/regulatory-information/search-fda-guidance-documents/
potency-tests-cellular-and-gene-therapy-products. Published
January 2011. Accessed December 13, 2023.
4. Salmikangas P, Carlsson B, Klumb C, Reimer T, Thirstrup S.
Potency testing of cell and gene therapy products. Front Med.
2023;10. doi:10.3389/fmed.2023.1190016
5. Pan K, Farrukh H, Chittepu VCSR, Xu H, Pan C, Zhu Z. CAR
race to cancer immunotherapy: From CAR T, CAR NK to CAR
macrophage therapy. J Exp Clin Cancer Res. 2022;41(1).
doi:10.1186/s13046-022-02327-z
6. McCune JS. Rapid advances in immunotherapy to treat
cancer. Clin Pharmacol Ther. 2018;103(4):540-544.
doi:10.1002/cpt.985
7. Albelda SM. CAR T cell therapy for patients with solid
tumours: Key lessons to learn and Unlearn. Nat Rev Clin
Oncol. 2023. doi:10.1038/s41571-023-00832-4
8. Daei Sorkhabi A, Mohamed Khosroshahi L, Sarkesh A, et
al. The current landscape of CAR T-cell therapy for solid
tumors: Mechanisms, research progress, challenges, and
counterstrategies. Front Immunol. 2023;14. doi:10.3389/
fimmu.2023.1113882
9. Morris EC, Neelapu SS, Giavridis T, Sadelain M. Cytokine
release syndrome and associated neurotoxicity in cancer
immunotherapy. Nat Rev Immunol. 2021;22(2):85-96.
doi:10.1038/s41577-021-00547-6
10. Xie G, Dong H, Liang Y, Ham JD, Rizwan R, Chen J.
CAR-NK Cells: A promising cellular immunotherapy for
cancer. eBioMedicine. 2020;59:102975. doi:10.1016/j.
ebiom.2020.102975
11. Liu E, Marin D, Banerjee P, et al. Use of CAR-transduced
natural killer cells in CD19-positive lymphoid tumors.
New Engl J Med. 2020;382(6):545-553. doi:10.1056/
nejmoa1910607
12. Klichinsky M, Ruella M, Shestova O, et al. Human chimeric
antigen receptor macrophages for cancer immunotherapy.
Nat Biotechnol. 2020;38(8):947-953. doi:10.1038/s41587-
020-0462-y
13. Lian X, Yang K, Li R, et al. Immunometabolic rewiring in
tumorigenesis and anti-tumor immunotherapy. Mol Cancer.
2022;21(1). doi:10.1186/s12943-021-01486-5
14. Guo C, Chen S, Liu W, et al. Immunometabolism: A new
target for improving cancer immunotherapy. Adv Cancer Res.
2019;143:195-253. doi:10.1016/bs.acr.2019.03.004
15. Zhang C, Liu J, Zhong JF, Zhang X. Engineering CAR-T cells.
Biomark Res. 2017;5(1). doi:10.1186/s40364-017-0102-y
16. Ramos CA, Dotti G. Chimeric antigen receptor (CAR)-engineered
lymphocytes for cancer therapy. Expert Opin Biol Ther.
2011;11(7):855-873. doi:10.1517/14712598.2011.573477
17. Grigor EJM, Fergusson D, Kekre N, et al. Risks and benefits
of chimeric antigen receptor T-cell (CAR-T) therapy in cancer:
A systematic review and meta-analysis. Transfus Med Rev.
2019;33(2):98-110. doi:10.1016/j.tmrv.2019.01.005
18. Melenhorst JJ, Chen GM, Wang M, et al. Decade-long leukaemia
remissions with persistence of CD4+ CAR T cells. Nature.
2022;602(7897):503-509. doi:10.1038/s41586-021-04390-6
19. European Medicines Agency. Potency testing of cell-based
immunotherapy medicinal products for the treatment of
cancer – scientific guideline. https://www.ema.europa.eu/
en/documents/scientific-guideline/guideline-potency-testingcell-
based-immunotherapy-medicinal-products-treatmentcancer-
revision-1_en.pdf. Published July 21, 2016. Accessed
December 13, 2023.
20. Food and Drug Administration. Draft Guidance Document:
Potency Assurance for Cellular and Gene Therapy Products.
https://www.fda.gov/regulatory-information/search-fdaguidance-
documents/potency-assurance-cellular-and-genetherapy-
products. Published December 2023. Accessed
January 23, 2024.
21. Alliance for Regenerative Medicine. Addressing potencyassay
related development delays for cell and gene
therapies: results of a scientific exchange between FDA and
developers. https://alliancerm.org/potency-assay-report/.
Published October 19, 2022. Accessed December 13, 2023.
22. Bravery CA, Carmen J, Fong T, et al. Potency assay
development for cellular therapy products: An ISCT*
review of the requirements and experiences in the industry.
Cytotherapy. 2013;15(1):9-19. doi:10.1016/j.jcyt.2012.10.008
23. Gómez-Cid L, Grigorian-Shamagian L, Sanz-Ruiz R, et al. The
essential need for a validated potency assay for cell-based
therapies in cardiac regenerative and reparative medicine. A
practical approach to test development. Stem Cell Rev Rep.
2021;17(6):2235-2244. doi:10.1007/s12015-021-10244-5
24. The European Parliament, The Council of the European Union.
Council Directive 2001/83/EC of the European Parliament and
of the Council of 6 November 2001 on the community code
relating to medicinal products for human use. 2001. OJ L311.
https://eur-lex.europa.eu/eli/dir/2001/83/oj. Updated January
1, 2022. Accessed December 13, 2023.
25. US Code of Federal Regulations. Biological Products: General,
Title 21 C.F.R., Chapter I, Subchapter F, Part 600. https://www.
ecfr.gov/current/title-21/chapter-I/subchapter-F/part-600.
Published September 11, 2014. Accessed December 13, 2023.
26. European Medicines Agency. ICH Q6B Specifications: test
procedures and acceptance criteria for biotechnological/
biological products – Scientific guideline. https://www.ema.
europa.eu/en/ich-q6b-specifications-test-procedures-andacceptance-
criteria-biotechnological-biological-productsscientific-
guideline. Published September 1, 1999. Accessed
December 13, 2023.
27. European Medicines Agency. Guideline on quality, non-clinical
and clinical requirements for investigational advanced therapy
medicinal products in clinical trials – Scientific guideline.
https://www.ema.europa.eu/en/guideline-quality-non-clinicaland-
clinical-requirements-investigational-advanced-therapymedicinal-
products-clinical-trials-scientific-guideline. February
21, 2019. Accessed December 13, 2023.
28. Betts MR, Koup RA. Detection of T-cell degranulation: CD107a
and b. Method Cell Biol. 2004;75:497-512. doi:10.1016/
s0091-679x(04)75020-7
29. Brunner KT, Mauel J, Cerottini J-C, Chapuis B. Quantitative
assay of the lytic action of immune lymphoid cells of
51Cr-labelled allogeneic target cells in vitro; inhibition by
isoantibody and by drugs. Immunology. 1968;14(2):181-196.
https://www.ncbi.nlm.nih.gov/pmc/articles/PMC1409286/.
Accessed December 13, 2023.
30. Karimi MA, Lee E, Bachmann MH, et al. Measuring
cytotoxicity by bioluminescence imaging outperforms the
standard chromium-51 release assay. PLoS ONE. 2014;9(2).
doi:10.1371/journal.pone.0089357
31. Kiesgen S, Messinger JC, Chintala NK, Tano Z, Adusumilli
PS. Comparative analysis of assays to measure CAR T-cellmediated
cytotoxicity. Nat Protoc. 2021;16(3):1331-1342.
doi:10.1038/s41596-020-00467-0
32. Dong Z, Cheng WA, Smith DL, et al. Antitumor efficacy of
BAFF-R targeting CAR T cells manufactured under clinicready
conditions. Cancer Immunol Immun. 2020;69(10):2139-
2145. doi:10.1007/s00262-020-02614-8
33. Castella M, Caballero-Baños M, Ortiz-Maldonado V, et al.
Point-of-care CAR T-cell production (ARI-0001) using a closed
semi-automatic bioreactor: experience from an academic
phase I clinical trial. Front Immunol. 2020;11. doi:10.3389/
fimmu.2020.00482
34. Capelli C, Cuofano C, Pavoni C, et al. Potency assays and
biomarkers for cell-based advanced therapy medicinal
products. Front Immunol. 2023;14. doi:10.3389/
fimmu.2023.1186224
35. Zhu J, Wang X, Xu X, Abassi YA. Dynamic and label-free
monitoring of Natural Killer cell cytotoxic activity using
electronic cell sensor arrays. J Immunol Method. 2006;309(1-
2):25-33. doi:10.1016/j.jim.2005.10.018
36. Abassi YA, Jackson JA, Zhu J, OConnell J, Wang X, Xu
X. Label-free, real-time monitoring of IgE-mediated mast
cell activation on microelectronic cell sensor arrays. J
Immunol Method. 2004;292(1-2):195-205. doi:10.1016/j.
jim.2004.06.022
37. Cellular impedance. Agilent. https://www.agilent.com/en/
technology/cellular-impedance. Accessed December 13, 2023.
38. Peper JK, Schuster H, Löffler MW, Schmid-Horch B,
Rammensee H-G, Stevanović S. An impedance-based
cytotoxicity assay for real-time and label-free assessment of
T-cell-mediated killing of adherent cells. J Immunol Method.
2014;405:192-198. doi:10.1016/j.jim.2014.01.012
39. Erskine CL, Henle AM, Knutson KL. Determining optimal
cytotoxic activity of human her2neu specific CD8 T cells
by comparing the CR51 release assay to the xCELLigence
system. J Vis Exp. 2012;(66). doi:10.3791/3683
40. Cancer immunotherapy: Agilent xCELLigence RTCA
handbook. Agilent. https://www.agilent.com/cs/library/
applications/appliction-cancer-immunotherapy-xcelligence-
5994-1303en-agilent.pdf. Published January 28, 2023.
Accessed December 13, 2023.
41. Cerignoli F, Abassi YA, Lamarche BJ, et al. In vitro
immunotherapy potency assays using real-time cell analysis.
PLoS ONE. 2018;13(3). doi:10.1371/journal.pone.0193498
42. Stock S, Benmebarek M-R, Kluever A-K, et al. Chimeric
antigen receptor T cells engineered to recognize the P329Gmutated
Fc part of effector-silenced tumor antigen-targeting
human IgG1 antibodies enable modular targeting of solid
tumors. J Immunother Cancer. 2022;10(7). doi:10.1136/jitc-
2022-005054
43. Glienke W, Dragon AC, Zimmermann K, et al. GMP-compliant
manufacturing of TRUCKs: CAR T cells targeting GD2
and releasing inducible IL-18. Front Immunol. 2022;13.
doi:10.3389/fimmu.2022.839783
44. Naeimi Kararoudi M, Likhite S, Elmas E, et al. Optimization
and validation of CAR transduction into human primary
NK cells using CRISPR and AAV. Cell Reports Method.
2022;2(6):100236. doi:10.1016/j.crmeth.2022.100236
45. Klapdor R, Wang S, Morgan MA, et al. NK cell-mediated
eradication of ovarian cancer cells with a novel chimeric
antigen receptor directed against CD44. Biomedicines.
2021;9(10):1339. doi:10.3390/biomedicines9101339
46. Tóth G, Szöllősi J, Vereb G. Quantitating ADCC against
adherent cells: impedance‐based detection is superior to
release, membrane permeability, or caspase activation
assays in resolving antibody dose response. Cytom Part A.
2017;91(10):1021-1029. doi:10.1002/cyto.a.23247
47. Pan L, Mora J, Walravens K, et al. 2022 White paper on recent
issues in bioanalysis: FDA draft guidance on immunogenicity
information in prescription drug labeling, LNP & viral vectors
therapeutics/vaccines immunogenicity, prolongation effect,
ADA affinity, risk-based approaches, NGS, qPCR, ddPCR
assays (part 3 – recommendations on gene therapy,
cell therapy, vaccines immunogenicity & technologies;
immunogenicity & risk assessment of biotherapeutics
and novel modalities; NAb assays integrated approach).
Bioanalysis. 2023;15(14):773-814. doi:10.4155/bio-2023-0135
www.agilent.com
RA45320.6538773148
For Research Use Only. Not for use in diagnostic procedures.
© Agilent Technologies, Inc. 2024
Printed in the USA, February 15, 2024
5994-7162EN
Download the Article for FREE Now!
Information you provide will be shared with the sponsors for this content. Technology Networks or its sponsors may contact you to offer you content or products based on your interest in this topic. You may opt-out at any time.