Cell Therapy: Clinical Applications, Manufacturing and Regulation
Listicle
Published: March 8, 2024
|
Laura Elizabeth Lansdowne
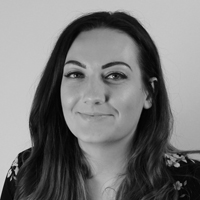
Laura Lansdowne is the managing editor at Technology Networks, she holds a first-class honors degree in biology. Before her move into scientific publishing, Laura worked at the Wellcome Sanger Institute and GW Pharma.
Learn about our editorial policies
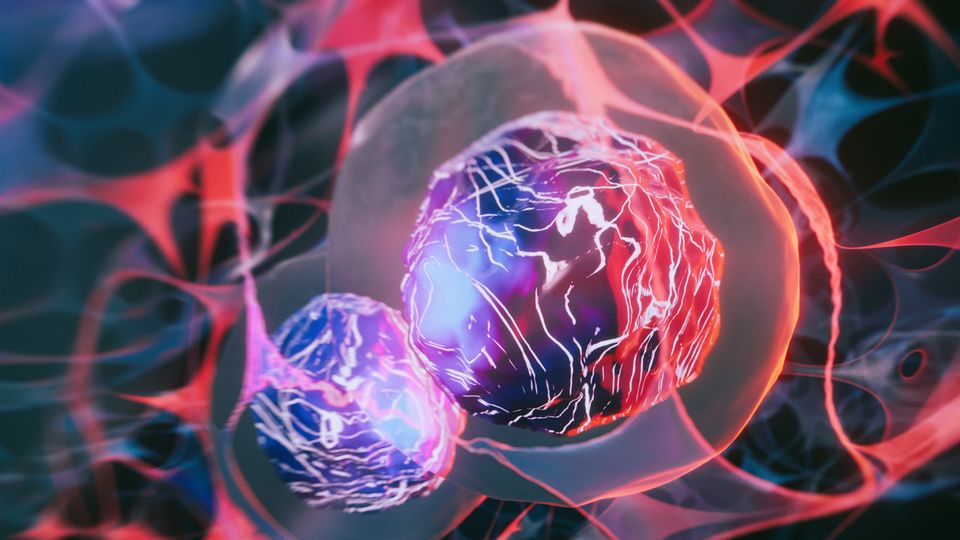
Credit: iStock
Cell therapies can be divided into stem-cell therapies and non-stem-cell therapies. Within these two groups, a variety of mechanisms of action are employed, tailored to the specific type of cell therapy and the disease or condition it’s designed to target.
The global market for cell therapy is expected to experience rapid growth due to increasing demand for innovative cell therapies. This, coupled with their wide range of clinical applications, is anticipated to propel the market to approximately USD 20 billion by 2030.
Download this listicle to learn more about:
- The diverse potential of cell therapy across different therapeutic areas
- The regulatory landscape of cell therapy
- Manufacturing and scalability challenges
CELL THERAPY: CLINICAL APPLICATIONS, MANUFACTURING AND REGULATION 1
Listicle
Cell Therapy: Clinical Applications,
Manufacturing and Regulation
Laura Elizabeth Lansdowne
Cell therapy is a therapeutic strategy that involves the transfer of autologous (patient-derived) or allogeneic (donorderived)
cells into a patient’s body. Cell therapies can be divided into two broad categories – stem-cell therapies and
non-stem-cell therapies. Within these two groups, a variety of mechanisms of action are employed, tailored to the specific
type of cell therapy and the disease or condition it’s designed to target.
For example, in regenerative medicine, stem cell therapies work by replacing damaged or diseased cells with new,
functional ones to restore organ and/or tissue function. Whereas adoptive cell therapies for cancer treatment exploit
immune cells, by either expanding the number of cells or by genetically modifying them to boost their cancer-fighting
abilities, before being administered to the patient. The three main cell therapy modalities are outlined in Figure 1.
In 2023, the global market value for cell therapy was estimated to be USD 4.74 billion. This market is expected to
experience rapid growth due to increasing demand for innovative cell therapies, which tend to have fewer adverse effects
compared to traditional modalities. This, coupled with their wide range of clinical applications, is anticipated to propel the
market to approximately USD 20 billion by 2030.
In this listicle, we explore new therapeutic targets and clinical applications, and discuss challenges and key considerations
related to the manufacture and regulation of cell therapies.
Cell Therapies
Cells are administered to a patient as a
therapeutic modality.
Genetically modified cell therapies
Patient/donor cells are genetically modified
to perform a unique function they wouldn't
typically do that provides therapeutic
benefit to a patient.
Tissue-engineered products
Cells and/or biologically active substances
are designed to restore, maintain or replace
damaged tissues/organs.
Figure 1: Overview of cell therapy modalities. Credit: Technology Networks.
CELL THERAPY: CLINICAL APPLICATIONS, MANUFACTURING AND REGULATION 2
Listicle
Clinical applications and novel research
While the oncology segment led the overall cell therapy market in 2023, the potential of cell therapy in other areas is
increasingly evident. Here we highlight recent research that illustrates the diverse potential of cell therapy across different
therapeutic areas.
Neurology
UC San Diego Health became one of the first facilities in the United States to administer the experimental neural cell
therapy, NRTX-1001, to subjects with drug-resistant epilepsy. This cell therapy involves the delivery of interneurons
that secrete the inhibitory neurotransmitter gamma-aminobutyric acid (GABA), into the epileptic region of the brain.
In December 2023, the biotherapeutics company developing NRTX-1001 announced that the initial two trial subjects
consistently reported a decrease in seizure frequency (> 95% reduction from baseline), more than one year after
receiving treatment.
Regenerative medicine
Using mRNA technology encapsulated in nanoparticles, researchers designed a novel stem cell therapy that aims to
stimulate the liver's natural repair processes. The study was published in Cell Stem Cell. The therapy works by
delivering vascular endothelial growth factor A (VEGFA) mRNA via lipid nanoparticles. This promotes the conversion
of biliary epithelial cells into hepatocytes, the liver's functional cells. The research was conducted using mouse and
zebrafish disease models.
Immunology
Researchers developed a novel T-cell therapy to treat a specific form of autoimmune encephalitis (NMDAR encephalitis),
an immune-mediated condition whereby antibodies attack healthy brain cells, causing inflammation and various
neurological symptoms. The team genetically modified T cells to selectively eliminate anti-NMDAR B cells and
autoantibodies against the NMDA receptor. This preclinical study was published in Cell. They now plan to test the therapy
in human subjects with NMDAR encephalitis.
Inherited blood disorders
The US Food and Drug Administration (FDA) approved two cell-based gene therapies – Casgevy™ (exagamglogene
autotemcel) and Lyfgenia™ (lovotibeglogene autotemcel) – to treat sickle cell disease in patients ≥ 12 years. Both
therapies are created using patients’ hematopoietic stem cells, which are genetically modified and then reintroduced
as a single-dose infusion. Casgevy is the first FDA-approved therapy that exploits CRISPR-Cas9 gene editing. CRISPRCas9
is used to reduce the expression of BCL11A, which in turn, boosts the synthesis of γ-globin and reactivates fetal
hemoglobin production, preventing sickling. Lyfgenia uses a lentiviral vector to genetically modify the stem cells to
produce a specific hemoglobin called HbAT87Q. Cells containing this hemoglobin have a reduced risk of sickling.
Oncology
A preclinical study published in Nature Communications describes a novel allogeneic CAR T-cell therapy targeting T-cell
malignancies. This therapy focuses on eliminating cancerous T cells that express a dominant T-cell receptor called
Vβ2. Unlike traditional treatments that risk depleting all of a patient's T cells, this CAR T-cell therapy selectively kills the
diseased cells, preserving Vβ2-negative healthy cells. CRISPR gene-editing technology was used to specifically target
the Vβ2-positive cancer cells.
The FDA approved Amtagvi™ (lifileucel), a tumor-derived autologous T-cell immunotherapy, via its Accelerated Approval
Program. Amtagvi is indicated for use in adult patients with a melanoma (unresectable or metastatic), that has failed to
respond to/stopped responding to other specific therapies. A portion of the patient’s tumor is surgically removed. TumorCELL
THERAPY: CLINICAL APPLICATIONS, MANUFACTURING AND REGULATION 3
Listicle
derived T cells are then isolated from the excised tumor tissue, expanded at a manufacturing site and then administered
as a single-dose intravenous infusion to the same patient.
Understanding the regulatory landscape of cell therapy
The pharmaceutical industry is one of the most regulated industries globally, and regulatory authorities play a crucial
role in overseeing various steps related to the development of new therapies. Several different regulatory authorities
exist worldwide and each issues specific guidelines relating to the development, registration, manufacturing, licensing,
marketing and labeling of medicines. As such, it is vital that cell therapy developers understand regulatory variations and
consider how these differences will impact the development of a novel cell therapy, depending on where in the world they
are seeking marketing authorization. Here we take a closer look at regulatory guidance in the UK, Europe and the US.
The European Medicines Agency (EMA) classes cell therapies as a type of advanced therapy medicinal product (ATMP).
As such, they are governed by medicinal product regulatory frameworks (Regulation (EC) No 1394/2007, Directive
2001/83/EC). The manufacturing of these products must comply with Good Manufacturing Practice (GMP) principles
(EudraLex Volume 4, Part IV).
Similar to the EMA, the UK’s Medicines and Healthcare products Regulatory Agency (MHRA) classes cell therapies as a
type of ATMP. The donation, procurement and testing of cells is covered by the EU Tissues and Cells Directive (2004/23/
EC). Under this directive, there are two authorities of note – the Human Fertilisation and Embryology Authority (HFEA) and
the Human Tissue Authority (HTA). The HFEA oversees the use of gametes and embryos in the development of ATMPs and
the HTA is responsible for the licensing and inspection for all other tissue and cell types.
In the US, cell therapies are regulated by the FDA’s Center for Biologics Evaluation and Research (CBER). They fall under
Title 21 of the Code of Federal Regulations (CFR), Part 127.3(d), and are defined as, “Articles containing or consisting of
human cells or tissues that are intended for implantation, transplantation, infusion or transfer into a human recipient".
The FDA recommends that “product testing for cellular therapies include, but not be limited to, microbiological testing
(including sterility, mycoplasma and adventitious viral agent testing) to ensure safety and assessments of other product
characteristics such as identity, purity (including endotoxin), viability and potency.” The FDA has numerous cellular and gene
therapy guidance documents, addressing specific aspects of cell and gene therapy development – for example, potency
assurance, manufacturing, trial design and general regulatory considerations.
Addressing manufacturing and scalability challenges
While the demand for cell therapies is undeniable, their production comes with key challenges and regulators require
manufacturers to conduct extensive testing. Cell therapy manufacturing methods range in complexity. Some therapies
require significant manipulation of cells (e.g., genetic modification), while others may require comprehensive cell
cultivation steps. Here we discuss several manufacturing and scalability considerations.
Sourcing high-quality cells
The initial challenge in cell therapy production lies in sourcing high-quality biological materials. The exact geographical
region and regulatory authority will influence how starting material must be obtained, for example collection/apheresis
best practices.
Progress is being made to refine the cell extraction and separation processes. For example, researchers recently
developed a technology capable of extracting mesenchymal stem cells (MSCs) directly from bone marrow – without the
need for dilution. By continuously sorting and isolating stem cells from blood cells using a novel microfluidic platform,
it was possible to double the number of MSCs obtained from bone marrow samples and reduce the extraction time to
approximately 20 minutes.
CELL THERAPY: CLINICAL APPLICATIONS, MANUFACTURING AND REGULATION 4
Listicle
In the case of allogenic (donor-derived) cell therapies, specific donor eligibility requirements will also need to be
considered as these may differ depending on country.
Establishing an appropriate shelf life
Once obtained, the shelf life of fresh cells is often short. For example, hematopoietic stem cells can be stored unprocessed
at 4 °C or room temperature for approximately 72 hours post-collection. However, after this time they begin to degrade,
resulting in compromised product quality and potency. To address this, developers typically opt to cryogenically freeze
cells, increasing their shelf life to months or years, but this relies on specialist facilities and procedures to ensure the
cells remain viable and stable. The optimal cryopreservation and freeze-thawing process will differ depending on the
particular cell product. Regulators require manufacturers to conduct stability testing to confirm the product's integrity and
efficacy over time.
Addressing temperature sensitivity
Increased temperature sensitivity is another key challenge faced by cell therapy manufacturers. Good distribution practice
guidelines stress that medicinal products must not be exposed to conditions during transport, “that may compromise
their quality and integrity.” Temperature changes must be tracked to confirm that products stay within "defined limits"
while in transit. The difficulty lies in establishing these limits, as they must be broad enough to allow transfer of the
product, but not so broad they jeopardize its quality.
Achieving quality bioproduction at scale
A product’s critical quality attributes (CQAs) must be well characterized early on in development before scaling
bioproduction to ensure the correct quality control metrics are being monitored. Developers want to avoid the need
for major manufacturing changes late in development as this could impact commercial viability of the therapy. The
term “technology transfer” describes the transfer of a process from small scale (a laboratory setting) to a commercial
manufacturing facility. If employing a contract manufacturing organization (CMO) or contract development and
manufacturing organization (CDMO) to support the scale-up of a therapy, it’s important to limit technology-transfer risk by
ensuring clear knowledge transfer, adequate training and ensuring equipment/process commonalities. Depending on the
type of cell therapy, developers will choose whether to scale-up (increase batch size) or scale-out (increase the number of
batches). Allogenic therapies are typically scaled up whereas autologous therapies are scaled out. Automated integration
of multiple manufacturing steps in a closed system environment is vital to ensure GMP compliance and process
reproducibility, and reduce contamination risk.
Conclusion
Continued exploration and investment in cell therapies is ushering in a new era of medical interventions. The discovery of
novel targets and mechanisms, refinement of manufacturing processes and creation of detailed regulatory guidance are
helping to accelerate the approval of cell therapies, offering hope and improved outcomes for patients worldwide.
Sponsored by
Sponsored by
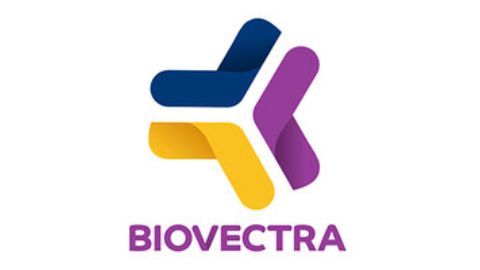
Download This Listicle for Free!
Information you provide will be shared with the sponsors for this content. Technology Networks or its sponsors may contact you to offer you content or products based on your interest in this topic. You may opt-out at any time.