An Introduction to Protein Purification: Methods, Technologies and Applications
Advanced protein purification techniques enhance protein activity and yield, facilitating breakthroughs in research.
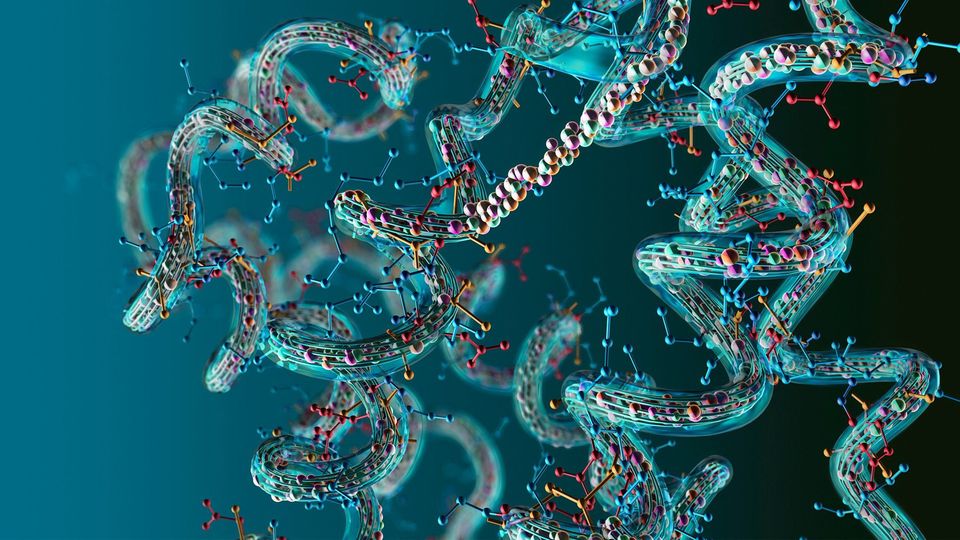
Complete the form below to unlock access to ALL audio articles.
Today, the ability to obtain pure proteins is essential for developing targeted drugs, creating vaccines and understanding biological processes at a molecular level. The development and optimization of protein purification methodologies have become increasingly important to meet the high demand for these proteins, ensuring they are free from contaminants that could affect their function. Advanced purification techniques enhance the yield and activity of proteins, facilitating breakthroughs in medical research and industrial applications. Continuous improvements in these methodologies enable more efficient, cost-effective and scalable production of high-quality proteins, driving innovation and discovery in numerous scientific domains.
What is a typical protein purification protocol?
Protein purification techniques
Recombinant protein expression
What is protein purification?
Protein purification is a process in molecular biology and biochemistry that involves isolating a specific protein from a complex mixture, often sourced from cells, tissues or other biological materials.1,2 This process is essential in many fields including:
- Biological research: to develop reagents like enzymes and antibodies that can be used as molecular biology tools for understanding cellular processes.3
- Diagnostics: purified proteins are used to develop assays and tests for diseases.4
- Environmental monitoring: protein-based biosensors are used to detect contaminants.5
- Food and cosmetics: protein content in food and cosmetic products must fulfill certain safety standards due to the risk of allergic reactions.6
- Forensic science: utilizes proteins for identifying substances in criminal investigations.7
- Biopharmaceutical development: purified proteins are pivotal for drug development and production, including therapeutic proteins and vaccines.8
What is a typical protein purification protocol?
A typical protein purification workflow involves several essential steps to isolate and purify the protein of interest while minimizing contaminants and maximizing yield and activity (Figure 1). This structured approach ensures the efficient isolation and detailed analysis of proteins.
1. Sourcing the protein
Proteins can be isolated from native tissues or cells where they are naturally expressed. This approach is often used for proteins that are difficult to express recombinantly or when studying proteins in their native context. Alternatively, many proteins are produced using genetically engineered organisms (see “Recombinant protein expression” section), allowing for high yields and easy manipulation of the expression.1,8
2. Extraction
The goal of this step is to break open cells to release their contents, including the target protein. It can be achieved by different approaches, such as mechanical disruption (commonly used for bacterial and yeast cells), chemical disruption (using detergents, organic solvents or chaotropic agents) and enzyme disruption (which can help break down cell walls, especially in bacterial cells). Freeze-thaw cycles and pressure cycling are alternative methods to lyse cells effectively. For further details, see the “Protein extraction methods” section. 1,8
3. Solubilization and stabilization
This step ensures that proteins remain soluble and stable in solution. This is achieved by employing appropriate buffers, protease inhibitors to prevent degradation and other agents to maintain protein solubility. For membrane proteins, detergents may be required to avoid their aggregation and/or precipitation. 1,8
4. Purification
Purification involves the isolation of the target protein from other cellular components. Some of the main techniques used for this goal are: i) Centrifugation: to separate cellular debris. ii) Precipitation methods: to concentrate proteins by altering solubility. iii) Chromatographic techniques (affinity, ion exchange, size exclusion, and hydrophobic interaction): to separate proteins based on different properties (molecular size, charge etc.). iv) Ultrafiltration and dialysis: to concentrate and desalinate samples, which is essential for downstream applications. For further details, see the “Protein purification techniques” section. 1,8
5. Characterization and analysis
This step is necessary to confirm the identity, purity and functionality of the purified protein. Some of the most common methods for the characterization and analysis of purified proteins are: i) Electrophoresis (Sodium dodecyl sulfate-polyacrylamide gel electrophoresis (SDS-PAGE)), which assesses purity and molecular weight. ii) Spectroscopic methods (UV-Vis, fluorescence), which determine concentration and structural properties. iii) Activity assays, which verify the functional integrity of the isolated protein. iv) Advanced techniques like nuclear magnetic resonance (NMR) spectroscopy, X-ray crystallography and mass spectrometry which provide detailed structural and functional insights. 1,8
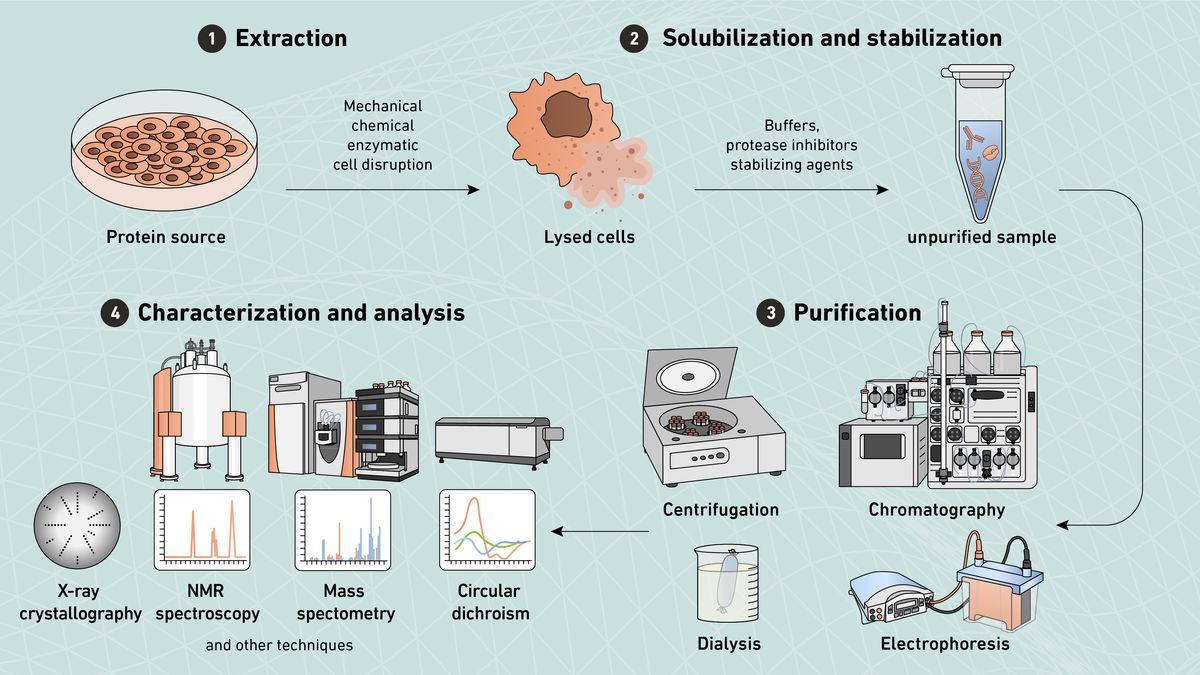
Figure 1: Workflow of a typical protein purification procedure. Credit: Technology Networks.
Protein extraction methods
Protein extraction is a crucial step in the purification process, involving the disruption of cells to release proteins. Various methods are used depending on the type of cells and the stability of the target protein (Figure 2). Each method has its advantages and limitations, which are discussed below:
1. Mechanical methods
Homogenization: This technique employs mechanical shear force to break open cells. It's highly effective for tough plant and animal tissues and it is scalable. However, the process can generate heat, which may denature sensitive proteins.9
Sonication: Utilizes ultrasonic waves to disrupt cell membranes. This is a quick approach commonly used for bacterial cells and it is very effective for small volumes. It requires cooling to prevent protein denaturation due to the generated heat.1
Pressure cycling: Consists of using high pressure to induce cell lysis and is appropriate for tough cells like yeast, being gentle on proteins. The disadvantage of this methodology is that it requires specialized equipment (a barocycler) which is not widely available.10
2. Non-mechanical methods
Detergents: Solubilize cell membranes by disrupting lipid bilayers. Some common detergents include Triton X-100 and SDS. This process is easy to use and very effective for membrane proteins, but it can cause denaturation if used at high concentrations. It may require removal before further purification. 1,8
Organic solvents: Solvents such as ethanol or acetone are used to precipitate proteins and disrupt membranes. It is a quick approach, but it is not suitable for all protein types, as it can cause denaturation. 1,8
Chaotropic agents: Disrupt the hydrogen bonding network in proteins, aiding in solubilization. Examples include urea and guanidine hydrochloride. This approach is especially useful for insoluble proteins, but can denature proteins and often requires subsequent refolding steps. 1,8,11
Freeze-thawing: This procedure involves repeated cycles of freezing and thawing to lyse cells by ice crystal formation. It is a simple way to lyse cells since it doesn’t require special equipment. However, it is time-consuming and not very efficient for some cell types. 1,8
Enzymatic treatment: Sometimes enzymes, such as lysozymes, are used to break down bacterial cell walls, in combination with other methods for enhanced efficiency. This approach is very specific and maintains protein integrity. Unfortunately, its use is limited to bacterial cells and requires additional steps for a complete cell lysis. 1,8
Each extraction method offers distinct advantages and limitations. The choice of method depends on the type of cells being used, the properties of the target protein and the subsequent purification steps planned.
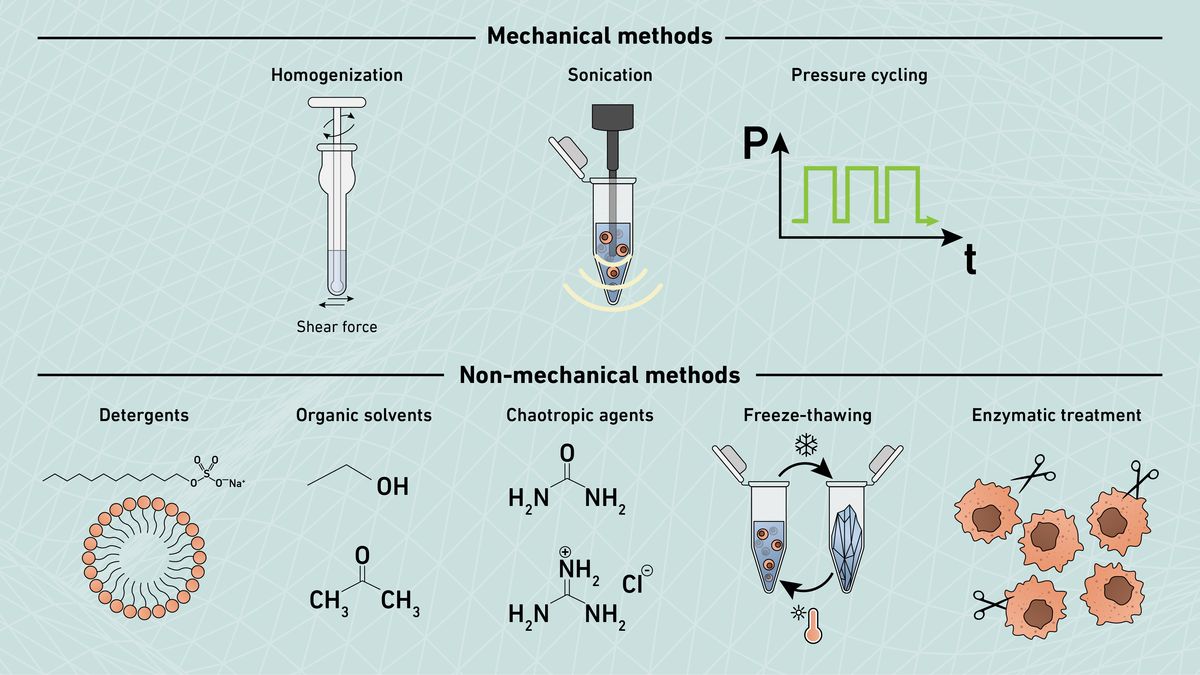
Figure 2: Commonly used protein extraction methods. Credit: Technology Networks.
Protein purification techniques
Protein purification involves several techniques, each tailored to isolate proteins based on specific properties like size, charge or affinity. Here’s a detailed look at each method:
1. Centrifugation
Centrifugation separates components based on their density by spinning samples at high speeds. During the process, heavier particles, such as cellular debris, sediment at the bottom, allowing the lighter supernatant, which contains the proteins, to be collected. This technique is often the first step in purification, used to remove large contaminants and concentrate proteins from crude extracts. 1,12
2. Precipitation
Precipitation involves altering the solubility of proteins to cause them to aggregate and precipitate out of solution. This can be achieved using salts (salting out), such as ammonium sulfate, organic solvents or changes in pH. Although this process can lead to the co-precipitation of contaminants, it is useful for the initial protein concentration and fractionation, particularly when working with large volumes. 1,13
3. Chromatography
Chromatography comprises of a varied set of versatile and widely used purification techniques that can be used to separate proteins based on various properties (Figure 3).
Affinity chromatography exploits specific interactions between a protein and a ligand attached to a resin. The target protein binds to the ligand, allowing other components to be washed away, followed by elution of the protein under specific conditions. This technique is suitable for proteins with known binding partners or tags (e.g., His-tagged proteins). 1,14
Ion exchange chromatography separates proteins based on their charge. Proteins bind to charged resins (cationic or anionic) and are eluted by increasing the ionic strength or changing the pH of the buffer. This is useful for proteins with well-defined charge, and it is often used as an intermediate purification step. 1,15
Size exclusion chromatography (SEC) separates proteins based on size by passing them through a column filled with porous beads. Smaller proteins enter the pores and elute later, while larger proteins bypass the pores and elute earlier. This technique is usually used for separating monomers from aggregates and as a final polishing of the protein purification process. 1,16
Hydrophobic interaction chromatography (HIC) separates proteins based on hydrophobicity. Proteins bind to hydrophobic groups on a resin at high salt concentrations and elute as the salt concentration decreases. This approach is appropriate for proteins containing highly hydrophobic domains and helps remove undesired aggregates. 1,17
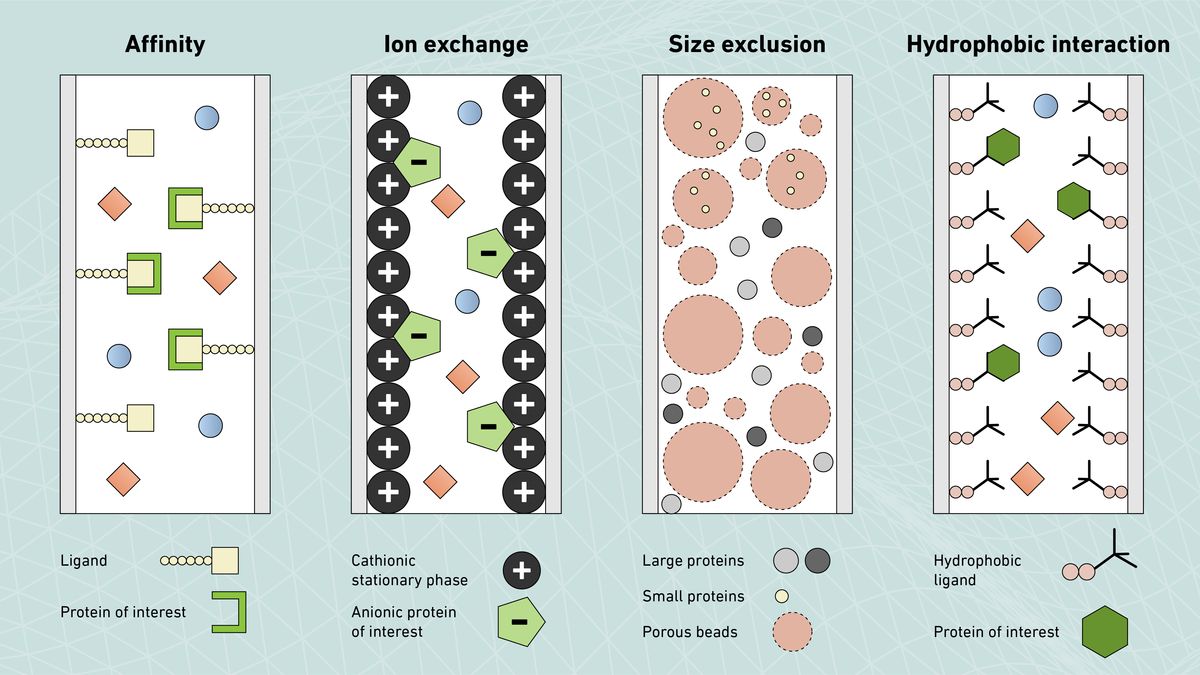
Figure 3: Scheme of the columns employed in some of the typical chromatography techniques used for protein purification. Credit: Technology Networks.
4. Ultrafiltration and dialysis
Ultrafiltration is a methodology that uses semipermeable membranes to concentrate and desalt protein solutions by applying pressure. 1,18 On the other hand, dialysis is a process that involves placing the protein solution inside a membrane with selective permeability, allowing small molecules to diffuse out to a surrounding solution. These methodologies are used to remove small contaminants, exchange buffers and concentrate proteins. 1,19
5. Electrophoresis
Electrophoresis separates proteins through a gel matrix based on size and charge by applying an electric field. One of the most common variants is SDS-PAGE, which uses a detergent (SDS) to denature proteins and separate them by size through a polyacrylamide gel matrix.20 Electrophoresis is both a technique for protein separation and the analytical assessment of protein purity and molecular weight determination.1
By understanding the strengths and limitations of each technique (see Table 1), researchers can choose the most appropriate methods for their specific protein purification needs, ensuring high purity and functionality of the target protein.
Table 1: Summary of protein purification techniques with their strengths, limitations and the most suitable samples.
Technique | Strengths | Limitations | Suitable samples |
Centrifugation | Simple, cost-effective, scalable | Not highly selective, may not remove small contaminants | Initial separation, crude extracts |
Precipitation | Cost-effective, rapid, simple | Can co-precipitate contaminants, requires optimization | Initial concentration, fractionation |
Affinity chromatography | High specificity and purity | Ligand binding needs optimization, expensive resins | Tagged proteins, specific binding partners |
Ion exchange chromatography | High resolution, scalable | Requires careful pH/ionic strength control | Proteins with defined charge properties |
Size exclusion chromatography | Gentle, useful for desalting and buffer exchange | Lower resolution, limited capacity | Final polishing, separating monomers/aggregates |
Hydrophobic interaction chromatography | Effective for hydrophobic proteins | Requires salt optimization, may denature proteins | Hydrophobic proteins, aggregate removal |
Ultrafiltration and dialysis | Simple, non-denaturing, cost-effective | Limited by membrane pore size | Concentration, buffer exchange |
Electrophoresis | High resolution, analytical | Typically, not preparative, can denature proteins | Purity assessment, molecular weight determination |
Recombinant protein expression
Recombinant proteins are genetically engineered to be produced by host cells that have been transformed with recombinant DNA. This DNA encodes the protein of interest, allowing the host cells to produce the protein in large quantities. The process of expressing recombinant proteins involves several steps (Figure 4):21,22
- Gene cloning: The gene encoding the protein of interest is isolated and inserted into a plasmid or other vector. This recombinant DNA construct often includes regulatory elements such as promoters and terminators to ensure proper expression.
- Transformation/transfection: The recombinant DNA is introduced into host cells. For bacterial and yeast cells, this process is called transformation, while for mammalian and insect cells, it is known as transfection.
- Selection: Host cells that have successfully incorporated the recombinant DNA are selected using antibiotic resistance markers or other selection systems encoded on the vector.
- Expression: The selected host cells are cultured under conditions that induce the expression of the recombinant protein. This step may involve optimizing growth conditions such as temperature, pH and nutrient supply.
- Harvesting: The host cells are lysed to release the recombinant protein, which is then purified using the aforementioned techniques.
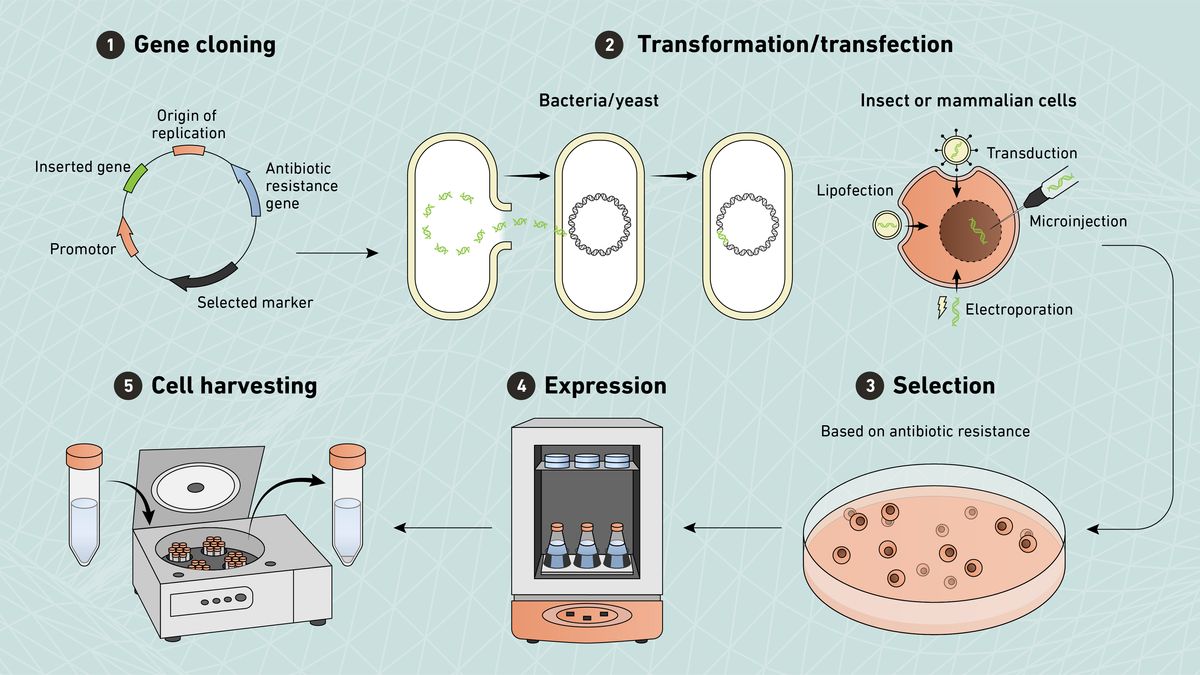
Figure 4: Workflow of a typical recombinant protein expression protocol. Credit: Technology Networks.
Different expression systems offer various advantages and limitations, making them suitable for specific applications. Table 2 summarizes the strengths and limitations of each system along with common applications:23,24
Table 2: Expression systems for recombinant proteins, including strengths, limitations, common applications and examples.
System | Strengths | Limitations | Applications | Examples |
Mammalian cells | Proper folding and post-translational modifications (PTMs), high biological activity | High cost, slower growth, complex culture requirements | Therapeutic proteins, complex glycoproteins | |
Insect cells | High yield, complex PTMs, easier culture conditions than mammalian | Moderate cost, PTMs may differ from mammals | Vaccine production, research proteins | Baculovirus expression systems27 |
Yeast cells | Fast growth, cost-effective, simple culture conditions | Limited PTMs compared to mammalian systems, hyperglycosylation | Industrial enzymes, basic research, vaccines | Hepatitis B vaccine production28 |
Bacterial cells | Rapid growth, high yield, simple and inexpensive culture | Misfolding, inclusion bodies, lack of PTMs, endotoxin contamination | Simple proteins, industrial enzymes | |
Algal cells | Sustainable, scalable, cost-effective | Less developed technology, variable protein yield | Biofuels, biopharmaceuticals, nutritional supplements | Algal chloroplast systems for vaccine antigens31 |
Cell-free | Rapid, scalable, no cell culture required, direct control over the environment | High cost, limited protein yield | Research, synthetic biology, high-throughput screening | Rapid prototyping of proteins32 |
Purification of recombinant proteins
Purifying recombinant proteins often requires specialized techniques to efficiently isolate the target protein while maintaining its functionality. Key methods include protein tagging, affinity purification and solubilization/refolding (Figure 5).33,34
1. Protein tagging and affinity purification
Protein tagging involves adding a specific amino acid sequence to the protein of interest to facilitate purification. The most common tags are His and GST tags, but there are others such as MBP, FLAG and Strep tags (see Table 3).22,35
His-tag protein purification
The His-tag consists of a sequence of six to ten histidine residues that bind strongly to metal ions such as nickel or cobalt. This binding is utilized in immobilized metal affinity chromatography. The His-tagged protein binds to a resin charged with nickel or cobalt ions. Contaminants are washed away, and the protein is eluted with imidazole or by lowering the pH.36
GST-tag protein purification
The GST-tag is a glutathione S-transferase enzyme that binds to glutathione immobilized on a resin. The GST-tagged protein binds to a glutathione resin and, after washing away contaminants, the protein is eluted with reduced glutathione.37
Table 3: Some common types of protein tags.38
Tag | Description | Binding partner | Size | Applications |
His | Polyhistidine sequence | Nickel/cobalt ions | Small | General purification, protein interactions |
GST | Glutathione S-transferase | Glutathione | Large | Solubility enhancement, activity assays |
MBP | Maltose-binding protein | Amylose | Large | Solubility enhancement, protein folding |
FLAG | Short peptide (DYKDDDDK) | Anti-FLAG antibody | Small | Western blotting, immunoprecipitation |
Strep | Streptavidin-binding peptide | Streptavidin | Small | Gentle elution conditions |
2. Solubilization/refolding
Sometimes, recombinant proteins form insoluble aggregates called inclusion bodies, especially in bacterial expression systems. Refolding and solubilization techniques are used to recover functional proteins. The procedure includes the solubilization of the inclusion bodies using chaotropic agents like urea or guanidine hydrochloride that denature the proteins. Then, the protein is slowly refolded by gradually removing the chaotropic agents, often in the presence of additives that assist in proper folding.39,40
Each purification approach for recombinant proteins has strengths and limitations (see Table 4) and researchers must select the most appropriate method to ensure the highest purity and functionality for the desired applications.
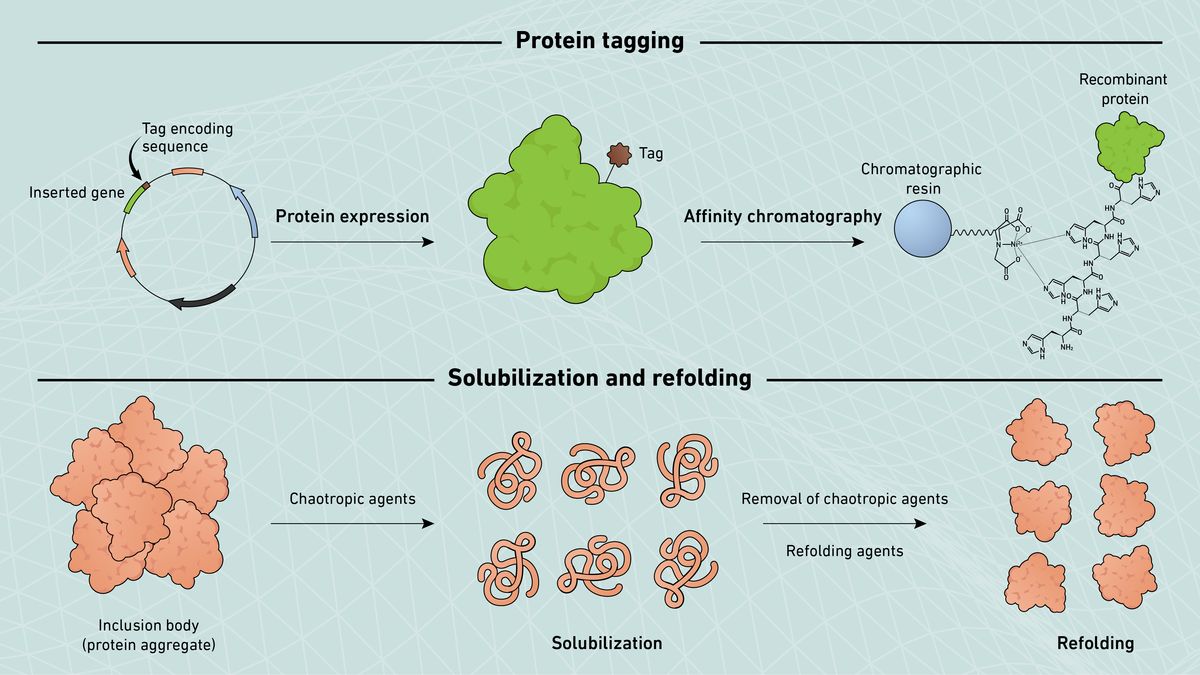
Figure 5: General scheme of the main methodologies for the purification of recombinant proteins. Credit: Technology Networks.
Table 4: Comparison of purification techniques for recombinant proteins.
Technique | Strengths | Limitations | Suitable proteins |
His-tag purification | Simple, robust, cost-effective | Potential metal leaching, may not bind all proteins | A broad range of proteins, especially those expressed in E. coli |
GST-tag purification | High affinity, enhances solubility | Large tag may affect function, requires tag removal | Proteins requiring enhanced solubility, eukaryotic proteins |
Refolding/solubilization | Recovers functional protein from inclusion bodies | Time-consuming, requires optimization | Insoluble proteins, proteins expressed in bacteria |
Protein analysis
After purifying proteins, analyzing their concentration, purity, activity, structure, stability and interactions is crucial. Here, we explore the most common techniques for protein analysis.
1. Protein concentration determination
Bradford assay: This colorimetric assay uses Coomassie Brilliant Blue dye, which binds to proteins, causing a shift in absorption. It's quick and straightforward but can be influenced by the presence of detergents.41
Bicinchoninic acid (BCA) assay: Another colorimetric assay, BCA assays measure the reduction of Cu2+ to Cu+ by proteins in an alkaline environment. It's compatible with detergents but less sensitive to protein composition variations.41
Fluorescence assays: These assays use fluorescent dyes that bind to proteins, providing high sensitivity and accuracy.41
UV-Vis spectrophotometry: This technique measures absorbance at 280 nm due to the presence of aromatic amino acids. It's non-destructive but less accurate for complex mixtures without a standard curve.42,43
2. Purity analysis
SDS-PAGE: This technique separates proteins based on size. It's highly effective for assessing purity and molecular weight.20
Western blotting: Following SDS-PAGE, proteins are transferred to a membrane and probed with specific antibodies. This technique is excellent for confirming the presence and purity of a target protein.44,45
Enzyme-linked immunosorbent assay (ELISA): ELISA uses antibodies to detect specific proteins.46 It's sensitive and quantitative, useful for detecting host cell proteins in biopharmaceuticals.47
3. Activity assays
ELISA: Measures protein activity by detecting functional interactions with antibodies or ligands.48
Radioligand assays: These assays involve radioactively labeled ligands to study binding interactions, offering high sensitivity.49
Surface plasmon resonance (SPR): Measures real-time binding interactions between proteins and ligands without labeling, providing kinetic data.50
4. Structural analysis
NMR spectroscopy: Provides detailed information about protein structure, dynamics and interactions. It’s excellent for small to medium-sized proteins but requires large amounts of protein and isotopic labeling.51,52
Cryo-electron microscopy (Cryo-EM): Determines high-resolution structures of large complexes in near-native states. It’s powerful but requires specialized equipment.53
Circular dichroism: Measures the differential absorption of left- and right-handed circularly polarized light, giving insights into secondary structure content.54
Fluorescence spectroscopy: Analyzes intrinsic and extrinsic fluorescence to study protein folding and conformational changes.55
X-ray crystallography: Provides atomic-resolution structures of crystallized proteins.56,57
5. Stability testing
Stability is studied by using differential scanning calorimetry, a technique that measures the heat change associated with protein unfolding, providing data on thermal stability and folding.58
6. Quantitative analysis
Mass spectrometry is the most employed technique to perform accurate quantitative analysis, including measurement of protein abundance, structural analysis, identification of protein structures, folding and interaction and detection of PTMs (such as phosphorylation and glycosylation).59,60
By leveraging these techniques, researchers can comprehensively characterize purified proteins, ensuring their suitability for various applications in research, diagnostics and therapeutics.
Protein purification is a critical step in biotechnology, pharmaceutical development and research, enabling the production of high-purity proteins for diverse applications. Utilizing advanced techniques and sophisticated analytical methods, scientists can isolate and thoroughly characterize proteins. This process ensures that proteins meet the necessary standards for efficacy and safety of the final product.