A High-Throughput Platform for Fluorescence Image-Based Neurite Outgrowth Analysis
App Note / Case Study
Published: June 6, 2024
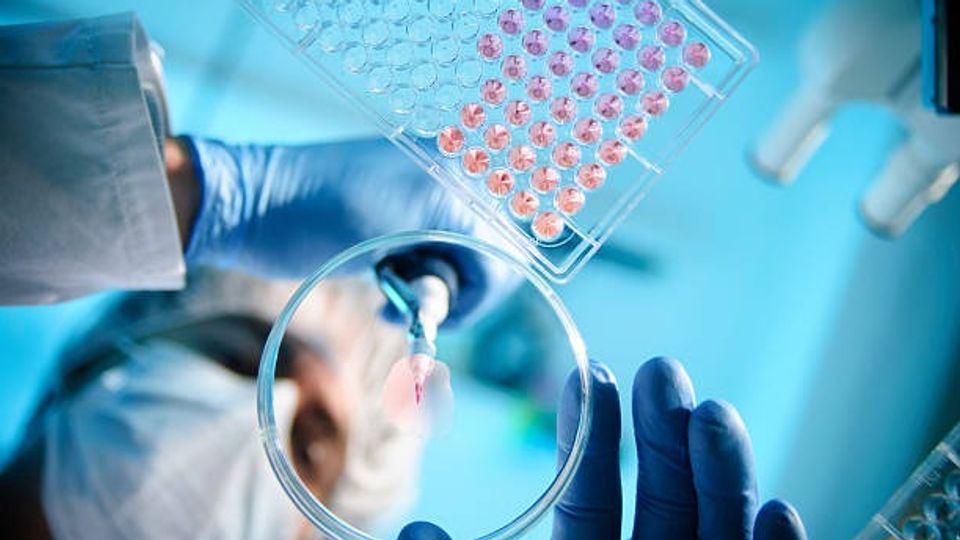
Credit: iStock
Image-based analysis is an essential tool for neurobiologists to evaluate neurite outgrowth as part of basic, translational and clinical research in neuroscience. Accurate assessment of neurite outgrowth is essential for understanding neurodevelopmental disorders, neurodegenerative diseases, and the effects of potential therapeutic compounds.
While a variety of imaging techniques can be used to evaluate neurite outgrowth, many workflows are labor-intensive, limiting throughput and flexibility.
This application note introduces an automated solution for flexible and sensitive multichannel analysis of neurite outgrowth for high-throughput assays.
Download this application note to explore how to:
- Automate sample preparation, image collection and analysis for both iPSC-derived and primary neuron culture models
- Detect dose–response effects across multiple parameters for neurite outgrowth assays
- Use a fluorescent immunocytochemistry-based approach to detect stimulatory and inhibitory effects
Application Note
Neuroscience Research
Authors
Rebecca Mongeon, PhD
Sarah Guadiana, PhD
Joe Clayton, PhD
Agilent Technologies, Inc.
Abstract
The evaluation of neurite outgrowth using high-throughput image-based analysis
provides an essential tool for neurobiologists to advance basic, translational, and
clinical research in neuroscience. In this application note, we introduce the Agilent
BioTek Gen5 neurite outgrowth module for flexible, automated, multichannel
analysis of neurite outgrowth. The module is compatible across Agilent BioTek
imaging instruments to provide a single platform solution for neurite outgrowth
assays. Neurite outgrowth analysis capabilities are demonstrated through proof-ofprinciple outgrowth evaluations for both iPSC-derived and primary neuron culture
models. Automated image collection and analysis was performed in a high-density
microplate format that enabled dose–response analysis of neuron cultures with
multiple neurite outgrowth characteristics. Fluorescent immunocytochemistrybased evaluations yielded sensitive and specific detection of both stimulatory and
inhibitory effects on outgrowth parameters in both neuron culture models.
High-Throughput Platform for
Fluorescence Image-Based
Neurite Outgrowth Analysis
Automated solution for quantitative neurite
outgrowth analysis of labeled iPSC-derived
and primary neuron culture models
2
Introduction
Neurons extend cellular outgrowths in a coordinated set of
developmental processes that serve to both establish and
maintain the cellular connections essential to the nervous
system’s communications network. These early outgrowth
processes, termed neurites, are developing dendrite and
axon compartments that will be responsible for incoming
and outgoing neurotransmission, respectively. In vitro neurite
outgrowth models have provided an essential platform for
determining the molecular and cellular mechanisms that
underlie both normal and aberrant neuronal development.1
Furthermore, neurite outgrowth plays a role in key neurological
health research areas focused on leveraging emerging stem
cell-derived models for developmental neurotoxicity testing,
neuroregeneration, and neurodegeneration.2-4
Models used for in vitro neurite outgrowth evaluations have
historically been acquired from primary murine tissues, and
immortalized neuronal-like cell lines typically derived from
malignant tissue sources. Although both model types have
been essential for foundational research, these models
present limitations for scalability, relevance, and predictive
power in neurological research.5
Comparatively, induced
pluripotent stem cell-derived (iPSC-derived) neuronal models
have a compelling profile for both translational and clinical
research owing to their established relevance and scalability.
Furthermore, iPSC-derived cell amenability to genome editing
techniques paired with the ability to apply human-derived
genetic backgrounds provide powerful handles for neuronal
disease phenotyping and personalized medicine.5
Neurite outgrowth is evaluated across neuronal culture
models using many imaging techniques, including label-free
methods, live-cell stains, membrane dyes, and antibody-based
immunofluorescence. Of these, antibody-based staining is
often relied upon for neuron detection, as it provides a level
of specificity that cannot be readily matched by alternative
methods. Antibody-based staining is clearly required for
neurite outgrowth analysis in complex culture models, such
as coculture or triculture model systems. However, specificity
can also be important for outgrowth in models where
neuronal differentiation is only occurring in a subpopulation
of cultured cells through addition or exclusion of media
factors.6,7 Primary cortical cultures also present mixed cell
populations that benefit from antibody-based analysis for
specificity, although tissue age and media components
can be leveraged to limit the presence and expansion of
nonneuronal cell populations.8
iPSC-derived neuronal cultures,
such as the iCell GlutaNeurons used in this study, can provide
a high degree of pure neuronal populations, but antibodybased evaluations are often still relied upon to establish
neuronal marker expression and outgrowth.9
The Agilent BioTek Gen5 neurite outgrowth module, used
with an Agilent BioTek cell imaging multimode reader,
provides a flexible and powerful system to support neurite
outgrowth research.This study demonstrates neuron culture
outgrowth analysis using advanced immunohistochemistry
techniques that focus on multiplexed fluorescent analysis of
two commonly used culture models for neurite outgrowth. We
detail how automation of sample preparation steps, image
collection, and image analysis can facilitate the sensitive,
high-throughput evaluation of neurite outgrowth while
minimizing user intervention and effort. Positive and negative
chemical modulators of neurite outgrowth are evaluated to
demonstrate the sensitivity and performance of the platform
to detect changes across multiple parameters relevant for
neurite outgrowth assays.
Experimental
Materials
Chemicals
All chemicals were purchased from Sigma unless otherwise
noted, including outgrowth effectors blebbistatin (p/n B0560),
6BIO (p/n B1686), and triptolide (p/n T3652). Staurosporine
was purchased from Tocris Bioscience (p/n 1285).
Cell culture
iCell GlutaNeurons (FUJIFILM Cellular Dynamics, Inc., p/n
R1061) cryopreserved iPSC-derived neurons were cultured
in BrainPhys Neuronal Medium (StemCell Technologies, p/n
05790) supplemented with iCell Neural Supplement B (iCell
kit component), iCell Nervous System Supplement (iCell kit
component), N-2 Supplement (Gibco, p/n 17502048), and
laminin (Sigma, L2020). Primary mouse cortical neurons
(Gibco, p/n A15586) were cultured in NeuroCult Neuronal
Plating Medium (StemCell Technologies, p/n 05713) and
supplemented with NeuroCult SM1 neuronal supplement
(StemCell Technologies, p/n 05711), GlutaMAX supplement
(Gibco, 35050061), L-glutamate (Sigma, p/n 1251), and
laminin (Sigma, p/n L2020). ICell GlutaNeuron and primary
mouse cultures were plated on Greiner glass bottom 96-well
microplates (Greiner, p/n 655892) for outgrowth assays.
Microplates for iCell GlutaNeuron cultures were first coated
with poly-L-ornithine solution (PLO: Sigma, A-004-M), followed
by complete media supplemented with laminin. Microplates
for mouse cortical neurons were coated with poly-D-lysine
(Gibco, p/n A3890401).
3
Immunohistochemistry
Anti-β-tubulin III antibody (β-tubulin) was purchased from
Sigma (p/n T8578) and anti-MAP2 antibody (MAP2) was
purchased from Invitrogen (p/n PA1-10005). Goat-anti-mouse
Alexa 633 secondary antibody was purchased from Thermo
Fisher Scientific (p/n A21052) and goat-anti-chicken Alexa
488 secondary antibody was purchased from Invitrogen (p/n
A11039). Bovine serum albumin (BSA) was purchased from
Sigma (p/n A3294).
Instrumentation
The Agilent BioTek Cytation 5 cell imaging multimode
reader with wide field of view camera was used for all image
acquisition and was outfitted with a 20x phase contrast
objective (p/n 1320517) and the following filter cubes: DAPI
(p/n 1225100), GFP (p/n 1225101), CY5 (p/n 1225105), and
LAF (p/n 1225010). The Agilent BioTek MultiFlo FX multimode
dispenser with the Agilent BioTek Automated Media
Exchange (AMX) module was used for plate washes between
immunohistochemistry steps. The Gen5 neurite outgrowth
module was used for all image analysis and data plotting.
Methods
Cell culture
Culture procedures followed manufacturer recommendations
for iCell GlutaNeurons and cryopreserved mouse cortical
neurons. Microplates were prepared for culture the day
before plating. Plates were first coated overnight at room
temperature with poly-L-ornithine (iCell GlutaNeurons) or
poly-D-lysine (mouse cortical neurons). Coated plates were
rinsed five times with sterile phosphate-buffered saline (PBS)
and incubated in a humidified tissue culture incubator (at
37 °C and 5% CO2
) for at least one hour before plating cells
with complete media (mouse cortical neurons), or complete
media plus laminin (iCell GlutaNeurons).
iCell GlutaNeuron and cryopreserved mouse cortical neurons
were thawed and plated following manufacturer protocol
recommendations. Live cell estimations were performed
with trypan blue exclusion method. Cells were plated at a
density of approximately 10,000 live cells per well. To promote
even cell dispersal across the well, plates were allowed to
rest at room temperature for approximately 30 minutes to
permit cell adhesion before transferring to a tissue culture
incubator. To minimize evaporative effects across the plate,
regions between wells were partially filled with sterile water.
For mouse cortical cultures, a 50% media exchange was
performed approximately two hours after initial plating.
After 24 hours of initial outgrowth, a dilution series of
outgrowth effectors were applied to both iCell GlutaNeuron
and mouse primary cortical culture in a 50% media exchange.
For iCell GlutaNeurons only, an additional 50% media
exchange was performed 48 hours later (72 hours following
plating) with maintenance of final drug concentrations.
Immunohistochemistry processing
After five days in vitro (120 hours), cultures were fixed with
4% paraformaldehyde for 15 minutes at room temperature.
Following fixation, 96-well microplates were washed with PBS
with 0.05% Tween-20 (PBST) using the MultiFlo FX with the
AMX module for five cycles (150 μL per cycle, 30 μL/second).
Cultures were permeabilized and blocked for one hour at
room temperature with a PBS solution containing 0.1%
TritonX-100 and 3% BSA. Permeabilized cultures were
incubated overnight at 4 °C with primary antibodies diluted at
1:5,000 in a PBST solution with 3% BSA. Following overnight
incubation in primary antibody, plates were washed five times
with the MultiFlo FX with AMX module. Secondary antibodies
were applied at a 1:500 dilution in PBST containing 1% BSA
and 1 μM DAPI nuclear counterstain and incubated for two
hours at room temperature. Following secondary antibody
incubation, plates were washed five times with a MultiFlo FX
with AMX module with PBST + 0.05% sodium azide.
Image capture and processing
Multichannel fluorescence images were captured at 20x
magnification in widefield mode in a 3 x 3 image montage
in the center of each well across the 96-well plate. Focus
was achieved using the laser autofocus method. Image tiles
were stitched to generate a single large image for neurite
outgrowth analysis. Stitched images were background
subtracted (100 μm rolling ball) before analysis for optimal
visualization of neuronal processes.
Image analysis
Default settings of the Gen5 neurite outgrowth module
provided a starting point for soma and neurite detection and
were further optimized as shown in Table 1. For best results
with immunolabeled cultures, it is recommended that both
immunostaining and nuclear staining signals are used for
soma detection. Optimal values for the parameters indicated
in Table 1 will vary based on experimental conditions, and
users are expected to evaluate and adjust these parameters
as needed.
4
Neurite Outgrowth Analysis Settings
Soma Detection iPSC-derived β-tubulin (CY5) and MAP2 (GFP) channels Mouse cortical culture β-tubulin (CY5) channel
Threshold Slider Level 20 30
Minimum/Maximum Size 10/100 10/100
Soma Closing Size 0 0
Optimize Soma Using Nuclear Signal Checked Checked
Rolling Ball Diameter 20 30
Nucleus Detection (DAPI Channel)
Threshold Slider Level 40 60
Minimum/Maximum Size 8/50 9.5/50
Minimum Nucleus/Soma Overlap 20 20
Maximum Nucleus/Soma Distance 5 8
Neurites
Threshold Slider Level Neutral Neutral
Neurite Mask Closing Size 0 0
Rolling Ball Diameter 10 15
Only Keep Neurites Connected to a Soma Checked Checked
Discard Short Neurites 10 10
Discard Short Ending Branches 5 8
Table 1. Neurite outgrowth analysis detection settings.
Results and discussion
Neurite outgrowth assay overview
Neurite outgrowth assays are relied upon across
neuroscience model systems to evaluate the characteristics
of neuron growth and extension of processes in both
compromised and healthy states, as well as in response
to drug treatment. In this study, two cell models frequently
evaluated through neurite outgrowth assays, mouse cortical
culture, and iPSC-derived neurons, were explored in a
proof-of-concept demonstration of the Gen5 neurite
outgrowth module.
Figure 1. Generalized workflow for immunofluorescence-based automated neurite outgrowth assays.
Data analysis and fitting
Gen5 microplate reader and imager software was used for all
data plotting and fitting. Dose–response relationships were
fit with four-parameter fits and Z-scores were calculated in
Gen5 using the difference between the treatment mean and
control mean expressed in multiples of the standard deviation
of control. In the Gen5 software syntax, the following formula
was applied in a transformation step to calculate the Z-score
for each outgrowth parameter: (X-MEAN(CTL1))/SD(CTL1)
5
A general neurite outgrowth assay schematic for
immunohistochemistry is shown in Figure 1. Cultures
were plated on high-density microplates and processed
for evaluation after five days of outgrowth. Multiplexed
immunohistochemistry was conducted using two markers
for neurite outgrowth and a nuclear counterstain. The
MultiFlo FX multimode dispenser with AMX was used for
gentle plate washes between immunohistochemistry steps
to both maximize efficiency and minimize potential damage
from manual pipetting. Multichannel fluorescent images
were automatically captured on the Cytation 5 cell imaging
multimode reader across the plate and analyzed with
Gen5 neurite outgrowth module. Multiple types of analysis,
including dose–response analysis shown in Figure 1,
can be performed in Gen5 software to evaluate treatment
effects on outgrowth.
Image collection and processing for neurite
outgrowth analysis
Image-based neurite outgrowth measurements used
multichannel fluorescent immunocytochemistry to evaluate
two neurite outgrowth markers and nuclear counterstain as
outlined in Figure 2. A 20x objective was used for all image
acquisition and images were tiled to sample a large region
for analysis (Figure 2A, left panel). Montages were stitched
together automatically to evaluate a representative central
FOV in the well (Figure 2A, center panel). Images were next
background subtracted to aid in visualization of neuronal
processes (Figure 2A, right panel). Background subtraction is
an optional step that is not required for analysis but can help
optimize visualization of neurites for display of the analysis
results. Background subtraction yielded relatively minor
improvements with these cultures as observed by comparing
Figure 2A center and right panels. Individual fluorescent
channels are shown in Figure 2B with a composite overlay.
In maturing neurons, MAP2 antibody labeling is primarily
localized to dendrites, whereas the β-tubulin antibody
labels both axonal and dendritic compartments. This
compartmentation can be appreciated in the overlay image
where MAP2-positive neurites are also β-tubulin positive,
but many more processes, potentially the developing axonal
compartments, are labeled only by the β-tubulin antibody.
Figure 2. Automated image capture, processing steps, and example
immunocytochemistry results. (A) 20x magnification images are
automatically captured in multichannel acquisition on an Agilent BioTek
Cytation 5 cell imaging multimode reader in widefield mode. A 3 x 3 image
panel is captured for each well (left panel) and images are automatically
stitched to generate a large image montage for analysis (center panel).
Optional background subtraction can be automatically applied to improve
the visualization of neurite processes (right panel). (B) Example multichannel
images of cryopreserved iPSC-derived neuron cultures after five days in
culture stained with DAPI nuclear marker (top left), MAP2 antibody (top
right), β-tubulin antibody (bottom left) and composite overlay image for all
channels (bottom right). Scale bars correspond to 200 μm.
6
Multichannel fluorescent image-based neurite
outgrowth analysis
Multichannel montaged images were processed for neurite
outgrowth analysis for both β-tubulin and MAP2 staining
stepwise as indicated in Figure 3. Gen5 software neurite
outgrowth module supports analysis on a single fluorescent
channel (e.g., neuron staining) or can combine a second
fluorescent channel (e.g., DAPI staining) to optimize detection.
For neurite analysis with immunolabeled cultures, it is
recommended that both nuclear staining and immunolabeling
are used together for optimal results (Figure 3A).
The combination of nuclear staining with immunolabeling
helps optimize soma detection in several aspects. First,
many neuronal antibodies, including both β-tubulin and MAP2
antibodies, strongly identify the perinuclear somatic region,
but are weak or absent in the nucleus itself. Nuclear labels
effectively fill these immunolabeling voids for improved
soma segmentation. Second, the Gen5 neurite outgrowth
module uses nuclear signals to help separate clusters of
neuronal somas and can therefore provide more accurate
soma counts.
Third, Gen5 neurite outgrowth module includes built-in
options designed to leverage nuclear signals to limit the
detection of nonneuronal nuclei through a minimum percent
overlap criteria between the nuclear and somatic staining
signals. Finally, nuclear staining provides a means of setting
a distance limit for how far the soma signal can extend from
the nucleus, termed the nucleus/soma distance in Gen5
neurite outgrowth module. This feature assists in defining
the boundary of where the soma ends and the neurite begins
and can be adjusted according to user requirements. Soma
identification, depicted in Figure 3B, was identified with this
purpose-built combination of Gen5 neurite outgrowth module
features to accurately identify viable neuronal somas for
analysis.
Following soma detection optimization, neurite detection
was next optimized as shown in Figure 3C. Gen5 neurite
outgrowth module neurite filtering features provide the ability
to include or disregard detected neurites according to several
criteria. First, users can opt to include only neurites that are
connected to soma on the image for analysis. Electing this
filtering option will discard fragmented neurites and neurites
that originate from soma that are not within the image. The
neurite skeletons displayed in Figure 3C were filtered to only
include neurites connected to somas. However, it should be
noted that the images shown in Figure 3C are only a portion
of the entire field that was analyzed, and it is expected that
some neurites displayed connect to soma that are not visible
within the displayed region.
Figure 3. Immunocytochemistry-based neurite outgrowth detection
optimization for soma and neurites. (A) Example images of fluorescent
antibody staining for β-tubulin and MAP2 antibodies with DAPI nuclear
counterstaining, as indicated. The displayed image is a subregion of the
entire montage for display purposes. (B) The same image from panel
A overlaid with soma detection results (yellow solid mask overlay) for
β-tubulin (left panel) and MAP2 antibody (right panel) staining channels.
Detected soma were filtered based on multiple criteria including nuclear
size, and minimal overlap between the soma stain and nuclear stain. (C)
Neurite analysis results for β-tubulin (left panel) and MAP2 antibody (right
panel) staining shown with both soma mask overlay (solid yellow fill) and
neurite skeleton overlay (left panel: cyan, right panel: magenta). Neurite
skeleton overlay displays were increased to three-pixel width for improved
visualization. Scale bars correspond to 200 μm.
7
Additional filtering options permit users to set minimal
requirements to include neurites for analysis, such as
minimal acceptable lengths for fragmented neurites,
neurites extending directly from a soma, and terminal
neurite branches.
Neuron outgrowth response to inhibitors and enhancers
of neurite outgrowth
As a proof-of-principle demonstration, outgrowth of
cryopreserved iPSC-derived neuron and primary mouse
cortical neuron cultures were evaluated in response to
treatment with four drugs previously reported as potential
enhancers or inhibitors of neurite outgrowth.9
Cells were
plated at a density of 10,000 live cells (determined via postthaw trypan blue cell counting) per well in a 96-well plate
format for outgrowth evaluation. For iCell GlutaNeurons,
a live cell percentage of approximately 65% was observed
at thaw and total live-cell yields were consistent with the
manufacturer estimates. For cryopreserved mouse ECNs, a
live cell percentage of approximately 50% was observed at
thaw, also in line with the manufacturer's estimates.
At 24 hours post-thaw (culture day 1), 50% of the neuron
culture media was removed and replaced with a dilution
series of each of the four drugs at the final concentrations
indicated in Figure 4A. After 48 hours of drug exposure
(culture day 3), an additional 50% media exchange was
performed for iCell GlutaNeurons (while maintaining final
drug concentrations), in alignment with the manufacturer's
recommendations for media refreshment schedule.
Media exchanges between culture days 1 and 5 were not
performed for mouse ECN culture, per media manufacturer's
recommendation.
Neuron cultures were fixed on culture day 5 and evaluated
for neurite outgrowth with fluorescent immunostaining
and nuclear staining as summarized in Figure 2. For these
cultures, detection settings were optimized to include cells
that met nuclear and soma minimum size criteria that are
consistent with characteristics of neurons that were viable
at the time of fixation. The Gen5 neurite outgrowth module
detection settings used are detailed in Table 1. Detection
parameters are flexible and intended to provide users the
ability to optimize analysis as desired across a range of
expected cell characteristics.
iPSC-derived neuron culture response to outgrowth
stimulation and inhibition
Neuron culture outgrowth in response to treatment was
first evaluated for iPSC-derived cultures. Results of the
Gen5 neurite outgrowth module analysis are automatically
reported for both image-level metrics (e.g., total outgrowth
length in the image), as well as cell-level average values (e.g.,
per-cell average neurite length). Analysis was performed
independently for each antibody, and outgrowth dose–
response curves for β-tubulin evaluation (Figure 4C) and
MAP2 staining evaluation (Figure 4D) are presented for
several outgrowth parameters.
For iPSC-derived neurons, responses for each drug were
consistent with previous reports of the ability of the drugs to
enhance (staurosporine and blebbistatin) or reduce (6BIO and
triptolide) neurite outgrowth.9
However, blebbistatin produced
a relatively small (approximately 20%) increase in total
outgrowth for iCell GlutaNeurons, compared to staurosporine.
Staurosporine promoted neurite outgrowth until the highest
concentrations tested, where significant cell death was
abruptly observed, consistent with previous reports.9
To
estimate the drug effect through fitting, the highest drug
concentration for staurosporine was omitted from the fourparameter fit.
As opposed to the two stimulatory drugs tested, both 6BIO
and triptolide demonstrated substantial reductions in neurite
outgrowth with typical four-parameter dose–response
relationships. In addition to reductions in total outgrowth
measured at the image-level, the average per-cell neurite
length, branch count, and neurite number were all reduced
with application of both 6BIO and triptolide (Figure 4C).
The results obtained with β-tubulin and MAP2 staining
evaluations (compare Figure 4C and D) largely indicated
general agreement on neurite outgrowth properties with
some exceptions. For triptolide, both staining evaluations
provided similar outgrowth inhibition and dose–response
characteristics. For 6BIO, however, β-tubulin staining indicated
a greater degree of inhibition compared to MAP2. It is
possible that this difference indicates that 6BIO has a greater
inhibitory effect on putative axonal outgrowth compared to
dendritic outgrowth.
When cell count values are consistent, total image level
outgrowth reflects the average outgrowth of any individual
cell. However, if cell numbers are changing, such as with loss
of neuron viability, it is often informative to also evaluate the
per-cell outgrowth average. In addition to image-level results
for total outgrowth, the Gen5 neurite outgrowth module
calculates the per-cell average neurite outgrowth values using
the soma count. For these inhibitory treatments, both the
per-cell average and image average outgrowth were in general
agreement, indicating the effect on outgrowth occurred at the
per-cell level and not only at the image level. This is consistent
with the specific inhibitory effect of 6BIO and triptolide on
neurite outgrowth and not simply a reflection of a general loss
of cell viability.
8
Figure 4. iPSC-derived neuron response to outgrowth enhancers and inhibitors. (A) Plate layout indicating dose–response curves for four drugs
tested in outgrowth assay. Plate view shows single image from montage of MAP2 immunofluorescence staining across the plate. (B) Automated
image analysis overlay display of the detected soma (yellow) and neurite skeletons (cyan) for three different example images of β-tubulin staining.
Scale bars correspond to 100 μm. (C) Dose–response curves for each treatment are plotted for four outgrowth metrics evaluated by β-tubulin
staining. Plots depict technical replicate mean (N = 3) and standard deviation for each condition. Four-parameter fit lines (dashed black lines) are
shown for each curve as indicated. Staurosporine treatment at the single highest concentration is omitted from fitting (open blue circle) to estimate
drug response. (D) Dose–response curves for each treatment are plotted for four outgrowth metrics evaluated by MAP2 staining. Plots depict
technical replicate mean (N = 3) and standard deviation for each condition. Four-parameter fit lines (dashed black lines) are shown for each curve as
indicated. Staurosporine treatment at the single highest concentration is omitted from fitting (open blue circle) to estimate drug response.
9
Cryopreserved mouse cortical culture outgrowth
response evaluation
Parallel investigation of outgrowth was undertaken for
cryopreserved primary mouse cortical cultures, and was
evaluated over the same time course, drug concentration
series, and immunohistochemistry approach. Figure 5A
shows example images of neurite outgrowth evaluated in
control wells and wells treated with positive and negative
effectors as indicated after five days of culture. To simplify
comparison against iPSC-derived cultures, neurite outgrowth
results are presented only for β-tubulin staining.
Blebbistatin produced a large increase in neurite outgrowth
in mouse cortical cultures, demonstrated by dose–response
evaluations across multiple parameters using both Gen5
neurite outgrowth module analysis (Figure 5B) and cellaveraged analysis (Figure 5C). Interestingly, blebbistatin
was the only drug that demonstrated a dose-dependent
increase in the number of somas (Figure 5B). As blebbistatin
inhibits myosin II implicated in both apoptosis and neuronal
outgrowth10, it is possible that blebbistatin is protective
against some of the apoptotic cell loss related to primary cell
culture cryopreservation.
Staurosporine, however, demonstrated a complex effect
in primary mouse neurons that differed from the relatively
robust and consistent outgrowth enhancement observed for
iPSC-derived neurons. Cell viability was reduced, as soma
counts, total outgrowth, and total branches all decreased with
increasing concentrations (Figure 5B). However, the cells that
did survive at higher staurosporine concentrations exhibited
a greater average number of outgrowths and branches,
concurrent with a shorter measured average length
(Figure 5C). This combination of effects suggests
staurosporine may promote neurite protrusion in this model,
but not overall length.
Although iPSC-derived neuron outgrowth was notably
inhibited by triptolide, outgrowth of mouse ECN-cultured
neurons was much less sensitive to triptolide as reflected
in the reduced effect size and comparatively shifted dose–
response curve across multiple measures of outgrowth
(Table 2). Although triptolide resulted in a relatively small
decrease in total neurite length at the highest concentrations
tested (Figure 5B), the average length and branching count of
the surviving cells was unchanged (Figure 5C); the absence
of average per-cell effects suggests a general decrease
in neuron viability that was consistent with the observed
decrease in soma counts (Figure 5B).
Comparative summary of iPSC-derived and primary mouse
neuron outgrowth
Table 2 provides a summary of the Gen5 software neurite
outgrowth module measurements for both iPSC-derived and
mouse neuron culture models. Control well averages are
indicated for each culture type, as well as percent effect size
(relative to control well values) and IC/EC50 values across
outgrowth metrics. For most treatments, outgrowth metric
responses were adequately fit with four-parameter dose–
response relationships and IC/EC50 values automatically
calculated in the Gen5 software for imaging and microscopy.
In some cases, however, plateaus for concentrationdependent effects were not well resolved. In those cases,
reported effect size and IC/EC50 values were instead
reported at 50% of the maximal response of the highest test
concentration using fit interpolation values in the
Gen5 software.
For each metric, Z-scores were calculated in Gen5 software
to determine whether treatment means were significantly
different from the control mean for each outgrowth
parameter. Outgrowth parameters with Z-score absolute
values greater than two across multiple consecutive
concentrations were considered to have a parameter mean
that was significantly different from control. Effect size and
IC/EC50 values are included for treatments with significant
responses in Table 2. Outgrowth parameters that did not
result in significant differences from control are indicated
with “ns.“
In addition to the default parameters measured by the
Gen5 neurite outgrowth module, an additional parameter
calculating the percentage of neurons that had outgrowth is
included in Table 2. For this percentage evaluation, only cells
that exhibited multiple (> 1) neurite counts were considered
to have significant outgrowth and included in the percentage.
This parameter was generated using the neurite module
“Group Neurons” functionality to count cells and create
subpopulations based on different available measurements.
Neuron grouping criteria are flexible to permit user-specified
groupings based on multiple parameters such as soma size,
signal intensity, or neurite count.
10
Figure 5. Cryopreserved mouse cortical culture response to effectors of neurite outgrowth. (A) Automated image analysis overlay displays the
detected soma (yellow fill) and skeletonized neurites (cyan lines) for three different example images of β-tubulin staining. Scale bar corresponds
to 300 µm. (B) Dose–response curves of neurite outgrowth parameters for β-tubulin staining evaluated at the image level, including soma count
(left panel), total neurite length (middle panel), and total neurite branches (right panel) for four drugs as indicated. Plots depict technical replicate
mean (N = 3) and standard deviation for each condition. For data series that conformed to a four-parameter fit, fit lines (dashed lines) are shown
for each curve as indicated. (C) Dose—response curves for neurite outgrowth parameters evaluated by per-cell average for neurite count (left
panel), average neurite length (middle panel), and average neurite branches (right panel). Plots depict technical replicate mean (N = 3) and
standard deviation for each condition. For data series that conformed to a four-parameter fit, fit lines (dashed black lines) are shown for each
curve as indicated. The highest concentration data point was omitted from staurosporine dose–response curve fitting as indicated (blue open
circles) to permit fitting over the remaining concentrations.
11
Neurite Outgrowth Summary
Untreated Staurosporine Blebbistatin 6BIO Triptolide
Value (%CV) Percent maximum relative change EC/IC50 (μM)
iPSCDerived
Mouse
Cortical
iPSCDerived
Mouse
Cortical
iPSCDerived
Mouse
Cortical
iPSCDerived
Mouse
Cortical
iPSCDerived
Mouse
Cortical
Soma Count 814
(11.7)
398
(6.7)
–82%
0.35 µM
–92%*
0.32 µM* ns + 29%
2.2 µM ns –25%
0.64 µM
–68%
0.0077 µM
–62%
0.11 µM
Total Neurite Length 257,000 μm
(11.6)
157,000 μm
(7.7)
+ 67%*
0.007 µM*
–62%*
0.001 µM*
+ 36%
0.26 µM
150%
2.3 µM
–50%
0.61 µM
–42%
0.75 µM
–95%
0.0076 µM
–70%
0.12 µM
Average Neurite Length 317 μm
(10.1)
395 μm
(8.6)
+ 61%*
0.1 µM* Complex ns + 94%
2.0 µM
–65%
0.4 µM
–22%
0.10 µM
–93%
0.011 µM ns
Total Neurite Branches 3472
(13.1)
1643
(6.6)
+ 64%*
0.01 μM* Complex ns + 42%
2.6 µM
–33%
2.2 µM ns –89%
0.0083 µM
–64%
0.21 µM
Average Neurite Branches 4.3
(7.8)
4.1
(7.5)
+ 57%
0.1 μM*
+ 75%
0.15 µM ns ns –50%
0.25 µM
+ 33%
0.56 µM
–84%
0.011 µM ns
Total Neurite Area 613,000 μm2
(8.4)
526,000 μm2
(7.1)
+ 31%
0.029 µM
–75%*
0.004 µM* ns + 112%
1.9 µM
–45%
0.92 µM
–44%
0.13 µM
–95%
0.0081 µM
–75%
0.15 µM
Neurite Thickness 2.4 μm
(4.3)
3.4 μm
(2.5) ns –31%
0.11 µM ns –15%
2.9 µM
+ 31%
0.27 µM ns + 29%
0.0069 µM
–19%
0.26 µM
Average Neurite Count 2.5
(4.1)
4.0
(6.5)
+ 19%
0.046 μM
+ 28%*
0.12 µM* ns + 34%
2.3 µM ns + 39%*
2.0 µM*
–75%
0.0087 µM
–36%
0.29 µM
Soma Size 13.2 μm
(1.4)
19.7 μm
(0.9)
–10%*
0.44 μM*
–30%*
0.34 μM* ns ns ns ns –10%
0.0046 µM
–23%
0.21 µM
Nucleus Size 9.2 μm
(1.2)
10.8 μm
(1.8) Complex ns ns ns + 8.7%*
0.14 µM ns + 6.6%
0.30 µM ns
Percentage Neurons
with Outgrowth
83.1%
(3.3)
91.4%
(2.6)
–85%
0.87 μM
–76%
0.87 μM ns + 6%
1.3 µM
–14%*
1.4 µM* ns –81%
0.0096 µM
–25%
0.29 µM
Table 2. Neurite outgrowth effect summary for iPSC-derived and primary mouse culture.
*Indicates that the effect size and EC/IC50 were estimated at 50% maximum/minimum response in cases where fit and response did not plateau.
“Complex” indicates that the effect was not monotonic.
Conclusion
Automated solutions for image-based neurite outgrowth are
essential to enable rapid and high-throughput neurobiology
investigations at scale. The Agilent BioTek Gen5 neurite
outgrowth module described here provides a flexible and
powerful solution that leverages the advantages of an
automated, high-throughput system for multimodal image
analysis. A key feature of the Gen5 neurite outgrowth module
for neurite outgrowth assays is the flexibility of multichannel
analysis across multiple fluorescence channels and
modalities to support a broad range of neurite
outgrowth approaches.
This study has provided a proof-of-principle demonstration for
neurite outgrowth analysis of both iPSC-derived and primary
mouse neuron culture models relevant to a broad range of
neurobiology research areas. We have focused on evaluating
these key neurite outgrowth models in a commonly used
immunohistochemistry-based approach to demonstrate the
usability and performance of the platform. Agilent BioTek
Gen5 microplate reader and imager software integrated the
automated image capture, processing, and neurite outgrowth
detection within a single interface and in a streamlined stepwise process. Data plotting and fitting capabilities of the Gen5
microplate reader and imager software readily transformed
the neurite image detection results into quantitative
analysis of the treatment effects. In both culture models
tested, significant dose–response effects were measured
across multiple parameters of neurite outgrowth for several
modulators of neuronal outgrowth.
References
1. Radio, N.M.; Mundy, W.R. Developmental Neurotoxicity
Testing in Vitro: Models for Assessing Chemical Effects
on Neurite Outgrowth. NeuroToxicology 2008, 29, 361-
376. https://doi.org/10.1016/j.neuro.2008.02.011
2. Blum, J; Masjosthusmann, S.; Bartmann, K.; Bendt, F.;
Dolde, X.; Dönmez, A.; Förster, N.; Holzer, A-K.; Hübenthal,
U.; Keßel, H.E.; et. al. Establishment of a Human
Cell-Based in Vitro Battery to Assess Developmental
Neurotoxicity Hazard of Chemicals. Chemosphere
2023, 311, 137035. https://doi.org/10.1016/j.
chemosphere.2022.137035
3. Al-Ali, H.; Beckerman, S.; Bixby, J.L., Lemmon, V.P. In Vitro
Models of Axon Regeneration. Exp Neurol 2017, 287, 423-
434. https://doi.org/10.1016/j.expneurol.2016.01.020
4. Logan, S.; Arzua, T.; Canfield, S.G.; Seminary, E.R.; Silson,
S.L.; Ebert, A.D.; Bai, X. Studying Human Neurological
Disorders Using Induced Pluripotent Stem Cells: From
2D Monolayer to 3D Organoid and Blood Brain Barrier
Models. Compr Physiol 2019, 9, 565-611. https://doi.
org/10.1002/cphy.c180025
5. Farkhondeh, A.; Li, R.; Gorshkov, K.; Chen, K.G.; Might,
M.; Rodems, S.; Lo, D.C.; Zheng, W. Induced Pluripotent
Stem Cells for Neural Drug Discovery. Drug Discovery
Today 2019, 24, 992-999. https://doi.org/10.1016/j.
drudis.2019.01.007
6. Radio, N.M; Breier, J.M.; Shafer, T.J.; Mundy, W.R.
Assessment of Chemical Effects on Neurite Outgrowth in
PC12 Cells Using High-Content Screening. Toxicological
Sciences 2008, 106-118. https://doi.org/10.1093/toxsci/
kfn114
7. Dravid, A.; Svirskis, D.; O’Carroll, S.J. Optimised
Techniques for High-Thoughput Screening of
Differentiated SH-SY5Y Cells and Application for Neurite
Outgrowth Assays. Sci Reports, 2021, 11, 23935. https://
doi.org/10.1038/s41598-021-03442-1
8. Blackmore, M.G.; Moore, D.L.; Smith, R.P.; Goldberg, J.L.;
Bixby, J.L.; Lemmon, V.P. High-Content Screening of
Cortical Neurons Identifies Novel Regulators of Axon
Growth. Mol and Cellular Neurosci 2010, 44, 43-54.
https://doi.org/10.1016/j.mcn.2010.02.002
9. Sherman, S.P.; Bang, A.G. High-Throughput Screen for
Compounds that Modulate Neurite Growth of Human
Induced Pluripotent Stem Cell-Derived Neurons.
Dis Model Mech 2018, 11, dmm031906. https://doi.
org/10.1242/dmm.031906
10. Wang, Y.; Xu, Y.; Liu, Q.; Zhang, Y.; Gao, Z.; Yin, M.; Jiang,
N.; Cao, G.; Yu, B.; Cao, Z.; et. al. IIA-Related Actomyosin
Contractility Mediates Oxidative Stress-Induced Neuronal
Apoptosis. Front Mol Neurosci 2017, 10, 75. https://doi.
org/10.3389/fnmol.2017.00075
www.agilent.com/lifesciences/biotek
For Research Use Only. Not for use in diagnostics procedures.
RA45404.3322222222
This information is subject to change without notice.
© Agilent Technologies, Inc. 2024
Published in the USA, May 15, 2024
5994-7380EN
Brought to you by
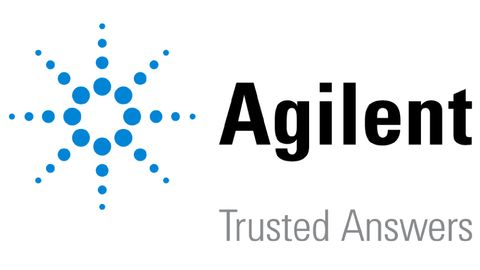
Download this App Note for FREE Now!
Information you provide will be shared with the sponsors for this content. Technology Networks or its sponsors may contact you to offer you content or products based on your interest in this topic. You may opt-out at any time.